This article critically examines the emerging skill gaps faced by professionals in PIC engineering and quantum computing, focusing on the strategic importance of continuing education strategies to maintain technological competitiveness and foster workforce development. It explores the intersection of PIC engineering and quantum technologies, analyzing how rapid advancements challenge traditional training frameworks and existing academic pipelines. Through an assessment of current credentialing schemes, industry-academia partnerships, and policy interventions, it identifies structural barriers that impede skill acquisition and transfer. The analysis emphasizes the need for targeted training programs, interdisciplinary curricula, and flexible credentialing models that align with evolving market demands. By offering a nuanced perspective on institutional cultures, psychological factors, and organizational incentives, the article proposes a roadmap toward sustainable skill-building approaches that leverage ongoing education, professional networks, and standardized competencies, ultimately accelerating innovation in quantum computing and PIC engineering domains.
Dr. Javad Zarbakhsh, Cademix Institute of Technology
Table of Contents
Introduction
The rise of quantum computing and the parallel evolution of photonic integrated circuit (PIC) engineering represent a critical juncture in the progression of cutting-edge technologies. As quantum computing professionals strive to implement increasingly complex photonic architectures, it becomes clear that the workforce must adapt swiftly. This adaptation is neither trivial nor guaranteed. Education and training pipelines, historically tailored to more conventional engineering and physics backgrounds, struggle to keep pace with the novel demands of integrated photonics and the quantum ecosystem. Rather than a seamless transition, many professionals find themselves confronting profound skill gaps, from fundamental optical design principles to advanced quantum device characterization.
At a strategic level, this reality raises pressing questions about continuing education strategies. In an environment defined by rapid innovations, the skill set required today will likely be outdated within a few years, if not sooner. The traditional approach, centered on formal degrees and lengthy academic programs, may prove too rigid and time-consuming. The workforce instead demands agile solutions—short courses, micro-credentials, industry-led training sessions, and integrated learning modules that respond dynamically to technology shifts. Policymakers, educational institutions, and industry leaders grapple with the challenge of balancing in-depth theoretical grounding with hands-on, application-focused training. Without a coherent framework, professionals risk stagnation, and organizations lose out on the promise of PIC-enabled quantum computing breakthroughs.
This article aims to dissect the complexities underlying the skill gap in PIC engineering for quantum computing professionals. It examines the multifaceted challenges at the intersection of technical complexity, educational inertia, and organizational culture. Drawing on industry reports, academic surveys, and policy documents, it identifies stumbling blocks and proposes strategic orientations. The analysis does not offer a simple solution; instead, it highlights structural barriers, encourages flexible credentialing schemes, and suggests ways to align professional development with the rapidly changing quantum landscape. In doing so, it seeks to guide stakeholders—engineers, educators, policymakers, and organizational leaders—toward more sustainable and adaptive continuing education strategies.
The Evolving Landscape of PIC Engineering in Quantum Computing
The allure of quantum computing lies in its potential to solve problems intractable by classical machines. Photonic integrated circuits (PICs), which guide and manipulate photons on chip-scale devices, are integral to achieving scalable quantum systems. As these circuits become more sophisticated, requiring precision engineering of waveguides, modulators, detectors, and nonlinear components, the skill requirements of professionals shift from a generalized understanding of optics or electronics to a nuanced mastery of quantum photonic integration.
This shift is not subtle. Traditional electronics engineers who might have dabbled in optical communication modules now face a new paradigm: designing quantum photonic chips that manipulate single photons, maintain quantum coherence, and integrate seamlessly with control electronics. Likewise, physicists specializing in quantum optics must learn the language of semiconductor fabrication, layout optimization, and PIC simulation tools. This cross-pollination of skills is intellectually stimulating but practically challenging. Without structured pathways, professionals often resort to ad hoc learning, depending on self-study, informal mentorship, or trial and error.
The complexity intensifies due to the rapid pace of innovation. Today’s cutting-edge PIC engineering techniques might become standard practice tomorrow, replaced by even more advanced fabrication methods, design software, or quantum protocols. Professionals must therefore embrace a philosophy of lifelong learning. Continuing education strategies should not be seen as an optional add-on to one’s career but as a core component of professional identity in the quantum computing domain. The workforce that thrives in this environment will be the one that normalizes ongoing skill acquisition, continuously retools its expertise, and responds fluidly to technological shifts.
Identifying the Skill Gaps: A Multifaceted Challenge
Before developing robust continuing education strategies, it is essential to understand precisely where the skill gaps lie. The technical domains of PIC engineering in quantum computing are extensive. They include waveguide design, nanofabrication, quantum state manipulation, device packaging at cryogenic temperatures, and integration with classical electronics and software stacks. Each area demands specific competencies. Yet, skill gaps are not merely a function of technical complexity; they often stem from organizational and cultural factors that make it difficult to acquire new skills efficiently.
For instance, educational curricula at universities frequently lag behind industry needs. While theoretical grounding in quantum mechanics and basic optics remains valuable, courses that cover state-of-the-art PIC simulation tools, semiconductor foundry processes, or specialized quantum device test protocols are less common. This mismatch leaves graduates well-versed in theory but underprepared for real-world applications. Moreover, the interdisciplinary nature of quantum PIC work means no single academic discipline provides a complete skill set. Physicists may lack engineering design principles, while electrical engineers may not fully grasp quantum state fidelity requirements.
Industry hiring practices and workforce development strategies may further exacerbate these gaps. Companies might prioritize immediate project deadlines over investing in training, thus expecting new hires to be “job-ready.” At the same time, professionals already in the field may struggle to find resources that fit into their demanding work schedules. The scarcity of tailored short courses, micro-credentials, and part-time academic programs compounds the challenge, leaving professionals to piece together skills from disparate and often inaccessible sources. Without systematic solutions, these skill gaps remain entrenched, inhibiting innovation and slowing the translation of quantum research into commercially viable products.
The Role of Continuing Education Strategies in Workforce Development
Continuing education strategies stand at the heart of bridging these skill gaps. Unlike traditional academic degrees, which demand full-time commitment and focus on foundational knowledge, continuing education is flexible, targeted, and modular. Short courses offered by universities, online platforms, or professional associations can provide critical updates on emerging PIC fabrication techniques or the latest quantum error correction schemes. Micro-credentials, such as industry-recognized badges or certificates, allow professionals to verify specific competencies without committing to an entire degree program.
These strategies must be informed by a thorough understanding of market dynamics and technological trajectories. For example, if integrated quantum photonics evolves toward silicon nitride platforms or adopts new inverse design algorithms for device layout, education providers must respond quickly. Rather than adjusting curriculum every few years, they must think in months or weeks, rapidly developing new course materials and integrating feedback from industry experts.
Continuing education also supports workforce diversification. As organizations seek talent from multiple domains—physics, engineering, computer science—continuing education provides a mechanism for individuals to cross-train and acquire missing competencies. This approach fosters interdisciplinary collaboration. Instead of relying on a small pool of highly specialized experts, organizations can build broad teams where each member continually upgrades their skill set. Such fluidity not only meets immediate technical needs but also cultivates an organizational culture that values learning, adaptation, and innovation.
Credentialing Schemes: Beyond Traditional Degrees
The concept of credentialing has long been associated with formal academic degrees or professional licenses. However, the fast-evolving nature of PIC engineering in quantum computing demands more agile credentialing schemes. Micro-credentials, digital badges, and stackable certificates represent new models that highlight specific skills or learning outcomes. They allow professionals to demonstrate competence in targeted areas, whether it’s mastering a particular PIC simulation tool or understanding device packaging constraints for quantum photonics.
This approach encourages continuous skill development. Instead of waiting two years to complete a master’s degree, professionals can earn credentials incrementally, reflecting immediate achievements and building confidence over time. This granular recognition of skills also supports employers. Hiring managers can look for candidates with a set of verified competencies relevant to current projects, rather than making do with vague academic transcripts or generic job experience claims.
Yet, challenges arise in standardizing credentialing schemes. Without common frameworks, credentials risk becoming meaningless badges without industry recognition. Establishing shared standards for PIC engineering competencies, endorsed by professional societies and industry consortia, would ensure consistency and credibility. If a micro-credential in “Quantum PIC Fabrication Techniques” is recognized uniformly across companies and geographic regions, it gains real value. Policy interventions, trade associations, and international collaborations can help build these standards, ensuring that credentialing fosters mobility, trust, and merit-based career advancement.
Industry-Academia Partnerships: Aligning Training with Market Needs
One of the most powerful strategies to bridge skill gaps involves closer collaboration between industry and academia. In the past, universities dictated curricula largely based on theoretical frameworks and broad educational goals. While this approach cultivates rigorous analytical thinking, it may not always produce graduates with the practical skills demanded by cutting-edge quantum computing environments. Industry partnerships can correct this imbalance. By sharing insights on emerging technologies, production constraints, and customer requirements, companies help academia stay up to date.
Such collaboration could materialize in several ways. Jointly developed training programs, co-taught courses, or internship pipelines allow students and professionals to gain hands-on experience with the tools and workflows that matter in real projects. Industry representatives might advise on course syllabi, ensuring that students learn about relevant PIC design software, device characterization instruments, or compliance standards. By the time learners enter the workforce, their skill sets more closely match industry expectations.
However, forging these partnerships is not always straightforward. Organizational cultures differ: universities prioritize knowledge dissemination and scholarly publications, while companies emphasize return on investment and speed. Overcoming these differences requires mutual respect, transparency, and willingness to negotiate. When successful, the synergy fuels a virtuous cycle: industry receives well-prepared talent, academia gains relevance and renewed funding streams, and professionals benefit from more coherent training pathways. Ultimately, these alignments make continuing education strategies more meaningful, reducing fragmentation and ensuring that training investments yield tangible results.
The Influence of Organizational Culture and Incentives
Skill gaps often persist not just because of technical complexity or inadequate educational programs, but also due to organizational cultures and incentive structures. In some workplaces, spending time on continuing education might be viewed as a distraction from immediate project deadlines. Managers might resist releasing employees for part-time study or investing in costly training courses, especially if benefits are not immediate or easily quantified.
To overcome this, organizations must embed skill development into their core values. Instead of treating continuing education as a luxury, they should recognize it as essential to long-term competitiveness. Incentive systems can encourage employees to pursue ongoing learning. Promotions, bonuses, or recognition programs can reward those who invest in updating their skill sets. If an engineer earns a new micro-credential in quantum PIC packaging, for example, this achievement should factor into performance evaluations and career progression.
Culturally, this shift depends on leadership. Leaders who publicly champion skill-building, budget for training, and personally participate in educational initiatives send a powerful message. When employees see top management investing in their growth, they are more likely to follow suit. Moreover, building communities of practice—internal networks where professionals share learning experiences, discuss new tools, or troubleshoot challenges—can reinforce a culture of continuous improvement. Over time, such cultures reduce resistance to change, lower skill gaps, and enhance collective knowledge.
Psychological Aspects: Overcoming Resistance and Burnout
Even if organizations and educational providers work together to create training opportunities, professionals may hesitate due to psychological factors. The rapid pace of technological change can be overwhelming. Some individuals may experience anxiety about falling behind or losing professional relevance, while others might resist learning new tools because they feel confident in their current competencies. Burnout is another risk; juggling job responsibilities, family commitments, and ongoing education can stretch individuals thin.
Addressing these psychological barriers is crucial. Professionals need supportive learning environments that acknowledge these pressures. Flexible training schedules, online courses that fit into busy lives, or modular learning paths help reduce stress. Mentoring and peer support groups can provide emotional backing, as colleagues share their struggles and successes in navigating continuing education. Encouraging a growth mindset—viewing learning as an ongoing journey rather than a series of isolated hurdles—further alleviates anxieties.
Policymakers and professional societies might offer guidance on work-life balance and mental health resources. If a training program demands significant time commitment outside of working hours, burnout risks increase. Designing short, focused learning modules or integrating training into the workday can help maintain motivation. Creating narratives that frame continuing education as an empowering step, rather than a burdensome obligation, is key. When professionals feel valued, seen, and supported, they are more likely to embrace skill development.
Interdisciplinary Curricula and Holistic Learning Approaches
As PIC engineering in quantum computing straddles multiple scientific and engineering domains, interdisciplinary learning becomes essential. A professional who excels in waveguide simulation but lacks an understanding of quantum state fidelity or cryogenic packaging techniques remains limited. Continuing education must therefore break down disciplinary silos, encouraging learners to explore beyond their comfort zones.
This holistic approach could manifest in various ways. A course on PIC design might also incorporate modules on quantum optics, device testing protocols, and data analysis methods relevant to quantum computing. Similarly, training programs could blend technical content with modules on project management, communication skills, or entrepreneurial thinking. Such breadth prepares professionals not only to solve current problems but also to adapt to unforeseen future challenges.
However, integrating multiple dimensions into a single curriculum is no small feat. Educators must carefully sequence topics to avoid overwhelming learners with information overload. They must curate materials that maintain depth while ensuring accessibility for professionals from different backgrounds. This balancing act might involve pre-assessment tests to tailor content to each participant’s baseline knowledge or offering optional modules that cater to diverse learning paths. When executed well, interdisciplinary curricula enrich the learning experience, foster creative problem-solving, and produce professionals capable of driving quantum PIC innovations from multiple angles.
Policy Interventions and Standardization Efforts
The complexity of skill gaps and continuing education strategies often calls for policy interventions. Governments, funding agencies, and professional bodies can incentivize educational reforms, support training initiatives, and facilitate the establishment of industry standards. For instance, granting agencies might require that large quantum computing projects include a skill-building component in their budgets, ensuring that knowledge transfer accompanies technological development.
Standardization efforts are equally important. Without commonly agreed-upon competencies, it is challenging to design curricula or credentialing schemes that gain industry-wide recognition. Professional societies or international committees could define a set of core skills for PIC engineering in quantum computing, publish guidelines for training programs, and create quality assurance mechanisms. When educational providers adhere to these standards, learners gain credentials that employers trust, reducing uncertainty in hiring decisions and career planning.
Policy interventions can also address regional inequalities. Not all professionals have equal access to advanced training facilities or well-funded institutes. Providing scholarships, travel grants, or online training platforms can broaden participation and ensure that the field does not become the domain of a privileged few. Over time, such equitable policies bolster workforce diversity, which in turn enriches the talent pool, encourages multiple perspectives, and drives more robust innovation.
Adapting to Technological Change: The Need for Agility
The quantum computing and PIC engineering sectors are moving targets. Just as educational programs catch up with one technology, a new breakthrough might render previous approaches outdated. Adopting agile strategies in continuing education is therefore vital. Agility implies faster curriculum development cycles, flexible pedagogical models, and a willingness to update course materials in response to user feedback and market shifts.
One possible solution involves continuous collaboration between educators and industry experts, who can provide timely insights on emerging trends. If a new PIC design methodology gains traction, training modules should reflect it within months, not years. Online platforms are well-suited to agile responses. They can update course content rapidly, track learner progress, and recommend new modules based on evolving skill sets.
Agility also means preparing learners to become self-directed. Instead of spoon-feeding knowledge, educational strategies can emphasize learning-to-learn skills—teaching professionals how to identify credible sources, evaluate new tools, and integrate knowledge independently. This meta-competence empowers individuals to stay ahead of the curve, reducing the dependency on formal courses for every new technological advance. In a rapidly evolving field, agility is as much about mindset and adaptability as it is about structural reforms.
Measuring Success and Ensuring Accountability
As with any strategic initiative, the effectiveness of continuing education strategies must be evaluated. Measuring success, however, is no trivial task. Traditional metrics—such as course completion rates or standardized test scores—may not fully capture the nuanced improvements in professional competencies or the long-term impact on innovation capacity.
More holistic approaches could involve tracking career trajectories: do professionals with certain credentials earn promotions more frequently, or do companies investing in continuous training see faster product development cycles? Surveys and interviews with learners can provide qualitative insights into their experiences, helping educators refine pedagogical methods. Case studies of successful PIC engineering projects can highlight how well-trained teams overcame technical challenges, linking educational investments to practical outcomes.
Accountability extends to funding sources and policy bodies. Those investing in skill-building want evidence that resources are used effectively. Periodic evaluations, benchmarking against international best practices, and open reporting of results foster transparency. Over time, these accountability measures create a feedback loop. Insights from evaluations inform future training programs, encourage continuous improvement, and ensure that the entire ecosystem—from educators to policymakers—remains committed to meaningful, measurable progress.
Cultural and Social Dimensions of Skill Development
In a field as specialized as PIC engineering for quantum computing, cultural and social dimensions are easily overlooked yet crucial. Training programs must cater to a global community, acknowledging language differences, cultural norms, and varying educational backgrounds. Some professionals might come from regions where photonics research is nascent, requiring more foundational training. Others might be native English speakers with easy access to online resources, giving them a head start.
Designing inclusive education means offering courses in multiple languages, providing accessible learning materials for people with disabilities, and acknowledging different learning styles. Mentorship programs can bridge cultural divides, pairing experienced engineers with newcomers from underrepresented backgrounds. Such an inclusive environment not only broadens participation but also enriches the knowledge ecosystem with diverse perspectives. In the long run, cultural sensitivity and inclusivity strengthen the workforce and encourage innovation by drawing on the full spectrum of human talent.
Social dynamics within organizations also matter. If a group of professionals pursues a training course together, they can form a supportive learning community, share insights, and reinforce new skills in daily work. Peer learning reduces isolation and motivates sustained efforts. Encouraging cross-team discussions about training experiences or hosting internal seminars where employees present new knowledge acquired from courses can institutionalize learning as a shared endeavor. In this way, social factors complement structural reforms, further enhancing the effectiveness of continuing education strategies.
Case Studies and Early Successes
While much of the discussion so far has been conceptual, some industry players and academic institutions have begun implementing elements of the strategies described. For example, a European quantum computing startup partnered with a local university to offer short intensive courses on PIC simulation tools. Engineers attended these courses on weekends, earning micro-credentials verified by the university and recognized by the startup’s human resources department. After a year, the company reported shorter development cycles and smoother integration of new hires, attributing improvements partly to better-trained staff.
In another scenario, a North American research consortium created an online platform where professionals could access lectures, practice labs, and discussion forums focused on quantum PIC packaging. Industry experts moderated these forums, answering questions and providing feedback. Over time, participants formed a virtual community that self-regulated quality, identified skill gaps, and requested new modules. The platform’s administrators used these inputs to refine courses, demonstrating how agile approaches and community involvement enhance relevance and effectiveness.
While anecdotal, these examples highlight that implementing continuing education strategies is not an insurmountable challenge. With foresight, collaboration, and investment, organizations can reap tangible benefits, nurturing professionals who are better prepared to tackle the complexities of quantum PIC engineering.
Risks of Inaction and the Cost of Missed Opportunities
Ignoring skill gaps or delaying the implementation of continuing education strategies carries risks. As quantum computing and PIC engineering advance, organizations that fail to upgrade their workforce’s skills may fall behind competitors who invest in training. This gap in competitiveness can have long-term consequences: missed market opportunities, inability to meet customer demands, or stagnation in research and development.
On a personal level, professionals might find their career prospects limited if they cannot adapt. In a field where cutting-edge skills determine who leads and who follows, those stuck with outdated expertise may face frustration, reduced job satisfaction, or, in extreme cases, involuntary job changes. Societally, a lack of skilled professionals in PIC engineering could slow the adoption of quantum computing technologies, depriving the public of potential benefits like faster drug discovery, secure communication, or optimized resource allocation algorithms.
The cost of missed opportunities should encourage stakeholders to act proactively. Investing in continuing education may have upfront costs—time, money, organizational effort—but these pale in comparison to the long-term disadvantages of falling behind. By confronting the skill gap now, stakeholders secure a foothold in a future defined by quantum computing innovations and integrated photonics breakthroughs.
Recommendations for Action and Future Directions
As the analysis suggests, bridging the skill gap in PIC engineering for quantum computing professionals is a multidimensional endeavor. Stakeholders should consider integrating multiple strategies:
- Educational institutions can create modular, interdisciplinary courses that combine theoretical and applied knowledge, updating content regularly.
- Industry should partner closely with academia, providing input on course design, funding training initiatives, and validating credentials.
- Professional societies can establish standards for competencies and accredit programs that meet these benchmarks.
- Policymakers can offer incentives for continuous learning, require skill-building components in grant applications, or provide funding for training facilities.
- Organizations should nurture cultures that value learning, providing incentives for employees to pursue credentials and supporting peer learning networks.
- Technological platforms can host agile, user-driven training materials and encourage feedback loops for continuous improvement.
Above all, the field must embrace a mindset that continuous education is not a temporary fix but a permanent feature of professional life. In the face of accelerating technological change, skill-building strategies must remain dynamic and context-aware, evolving alongside the quantum computing landscape.
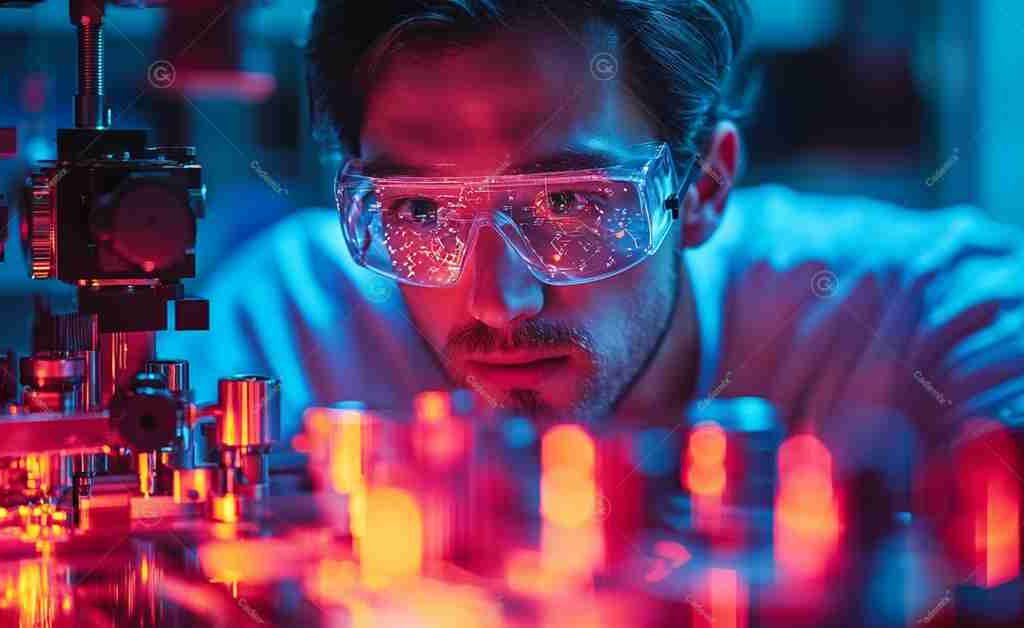
Conclusion
The skill gap in PIC engineering for quantum computing professionals is both a challenge and an opportunity. The complexity of integrated photonics and the rapid development of quantum technologies necessitate ongoing education. Traditional academic and corporate training models are inadequate for this rapidly shifting terrain. Without strategic interventions, skill gaps will persist, threatening competitiveness, innovation capacity, and the long-term success of quantum computing industries.
A call to action is clear: stakeholders must collaborate to design flexible credentialing schemes, agile curricula, and supportive organizational cultures. By recognizing that skill-building is a continuous process—not a one-time event—professionals, educators, and leaders can ensure that the quantum computing workforce remains at the forefront of innovation. The path forward involves thoughtful policy decisions, stable industry-academia partnerships, and the courage to experiment with new educational paradigms. If these efforts are sustained, the quantum computing community can look toward a future in which PIC engineering expertise thrives, fueling breakthroughs that transform industries and societies.
References and Further Reading
Bogaerts, W., & Chrostowski, L. (2018). Photonic integrated circuit design automation: Past, present, and future. IEEE Nanotechnology Magazine, 12(2), 20–29.
https://doi.org/10.1109/MNANO.2018.2819409
Flamini, F., Spagnolo, N., & Sciarrino, F. (2018). Photonic quantum information processing: A review. Reports on Progress in Physics, 82(1), 016001.
https://doi.org/10.1088/1361-6633/aad5b2
Nielsen, M. A., & Chuang, I. L. (2010). Quantum Computation and Quantum Information: 10th Anniversary Edition. Cambridge University Press.
https://doi.org/10.1017/CBO9780511976667
Perez, D., Gasulla, I., & Capmany, J. (2017). Field-programmable photonic arrays. Optics Express, 25(3), 282–294.
https://doi.org/10.1364/OE.25.000282
Politi, A., Matthews, J. C. F., Thompson, M. G., & O’Brien, J. L. (2009). Integrated quantum photonics. IEEE Journal of Selected Topics in Quantum Electronics, 15(6), 1673–1684.
https://doi.org/10.1109/JSTQE.2009.2026060
Soref, R. (2010). The past, present, and future of silicon photonics. IEEE Journal of Selected Topics in Quantum Electronics, 16(1), 167–176.
https://doi.org/10.1109/JSTQE.2009.2035196
Wang, J., et al. (2018). Multidimensional quantum entanglement with large-scale integrated optics. Science, 360(6386), 285–291.
https://doi.org/10.1126/science.aar7053
Xu, X., et al. (2020). Photonic inverse design: from fundamentals to applications. Advanced Optics and Photonics, 12(3), 828–887.
https://doi.org/10.1364/AOP.381966
Zhang, H., et al. (2021). Integrated quantum photonics: current status and future prospects. APL Photonics, 6(7), 071101.
https://doi.org/10.1063/5.0045024
European Quantum Flagship. (2021). Strategic Research Agenda for Quantum Technologies.
https://qt.eu/roadmap