This article provides an educational overview of how Lumerical and Synopsys simulation tools can be leveraged to design and analyze quantum-enhanced photonic integrated circuits (PICs), building on concepts that originated with photonic crystals. It explores the technical, organizational, and cultural dimensions that shape PIC simulation workflows, emphasizes the importance of material and fabrication constraints, and offers insights into bridging theoretical principles with practical implementation. While highlighting the complexity and sensitivity of quantum-enhanced circuits, it encourages learners to approach simulation as part of a broader design process involving iterative experimentation and critical evaluation. The discussion underscores the need for interdisciplinary collaboration, standardized practices, and policy support to accelerate the transition from conceptual designs to scalable, reliable quantum photonic architectures.
By: Javad Zarbakhsh, PhD, Cademix Institute of Technology
Table of Contents
Introduction
The evolution of integrated photonics, which once seemed destined to remain a niche technology employed only in advanced communications systems, has taken on renewed significance amid the growing interest in quantum-enhanced circuits. As the quest for scalable quantum computing and secure quantum communication intensifies, integrated photonics offers an enticing platform to guide, manipulate, and measure photons with unprecedented precision. Historically, the study of photonic crystals represented one of the earliest attempts to control light at the nanoscale, leveraging periodic dielectric structures to craft photonic bandgaps and shape the flow of photons much as semiconductors control electrons. Yet, as the field progressed, researchers gravitated from these early periodic lattices toward more compact and practical photonic integrated circuits (PICs) that use optical waveguides and advanced simulation techniques to design devices with complex functionalities.
This shift in focus has created a need for educational resources that not only delve into the fundamentals of photonic crystals and PIC components, but also guide engineers, physicists, and students through the simulation workflows needed to turn theoretical concepts into working prototypes. Within this ecosystem, tools such as Lumerical and Synopsys have emerged as industry standards for PIC simulation, enabling designers to model optical waveguides, analyze coupling efficiencies, evaluate mode profiles, and predict the performance of intricate quantum-enhanced circuits. Yet these tools can appear daunting to newcomers. Their comprehensive interfaces, broad feature sets, and abundant parameter spaces can deter learners who may find it challenging to move from photonic crystal theory to practical PIC design workflows.
The purpose of this article is to bridge that gap. Rather than focusing solely on the underlying physics, we aim to provide educational insights into how Lumerical and Synopsys tools can be applied to the modeling and simulation of quantum-enhanced PICs, using concepts that trace back to photonic crystals. The goal is not merely to give step-by-step instructions—though guidance will be offered where possible—but to highlight key considerations, conceptual linkages, and common challenges encountered in the simulation environment. By demonstrating how theoretical constructs from photonic crystals inspire PIC design, and then showing how to implement such designs in Lumerical and Synopsys, this article hopes to equip readers with a more holistic perspective of integrated photonics.
Throughout the discussion, we will return frequently to the idea that PIC simulation is not just about pressing buttons in a software interface. Instead, it demands critical thinking, attention to fabrication constraints, and awareness of the organizational and cultural factors that influence technology transfer from simulation to production. Moreover, we will emphasize challenges, acknowledging that designing quantum-enhanced circuits is far from trivial and that simulation tools, while powerful, do not eliminate the difficulties of scaling quantum photonic technologies. By doing so, we hope to set realistic expectations for what Lumerical, Synopsys, and similar PIC simulation tools can achieve, while fostering an environment that encourages further learning and exploration.
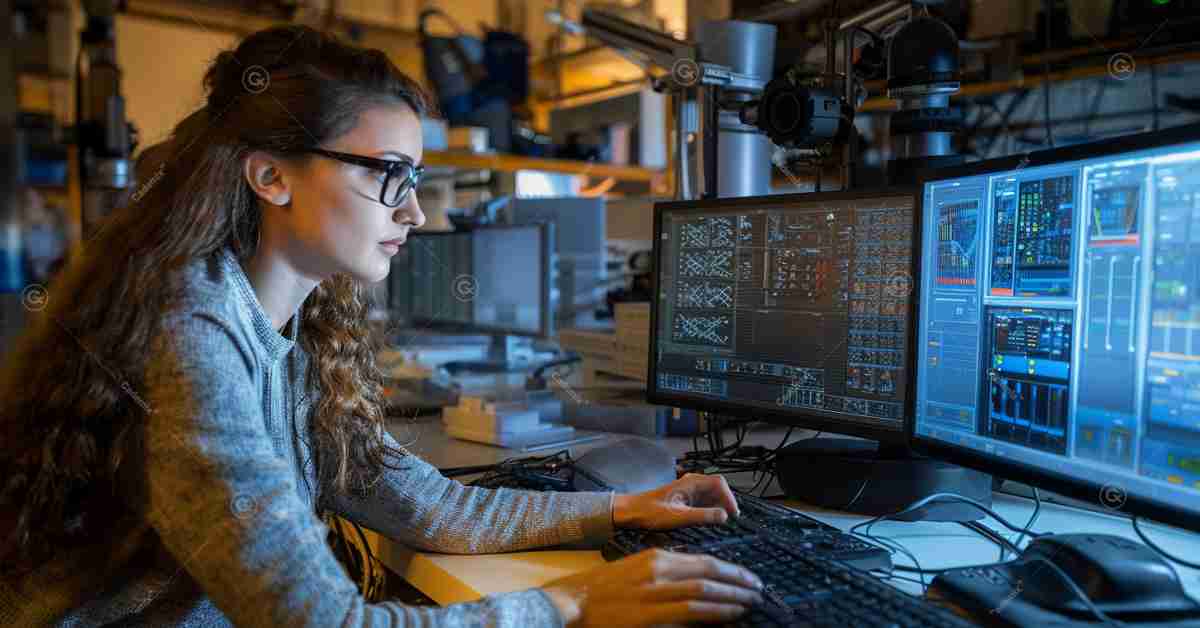
Photonic Crystals: Foundational Concepts and Their Limitations
Before diving into PIC simulation tools, it is helpful to understand the conceptual underpinnings offered by photonic crystals. Much like electronic band structures in solids, photonic crystals use periodic variations in refractive index to create frequency bands where light propagation is either allowed or forbidden. By tuning parameters such as lattice constant, hole size, or refractive index contrast, researchers could engineer photonic bandgaps that confine or route light in ways unattainable with uniform dielectric media. Early proofs of concept showed that photonic crystals could support waveguides and resonant cavities with high quality factors, making them attractive candidates for optical filtering, low-threshold lasers, and even quantum information processing.
Yet, despite these promising capabilities, photonic crystals have been challenging to scale into robust, manufacturable devices suitable for complex quantum-enhanced circuits. One of the primary reasons lies in the sensitivity of photonic crystals to fabrication imperfections. Achieving perfect periodicity and smooth interfaces at the nanoscale is nontrivial, and even small deviations can alter bandgaps, introduce scattering losses, or degrade device performance. Additionally, photonic crystal structures often require intricate geometries that may be incompatible with large-scale semiconductor foundry processes. These fabrication barriers limit the reproducibility and yield of photonic crystal devices, which, in turn, hinders the path to commercialization and large-scale integration.
From an educational perspective, photonic crystals remain valuable as conceptual tools. They reinforce the fundamental idea that light can be manipulated by nanoscale structuring of materials and that periodicity can yield strong confinement and mode engineering. These insights form the bedrock upon which modern PIC simulation builds. In a sense, PICs can be viewed as a more mature and scalable extension of photonic crystals’ initial vision. Rather than relying exclusively on periodic lattices to achieve desired functionalities, PIC designers leverage well-defined waveguides, couplers, modulators, and detectors. The move from photonic crystals to PICs involves a shift in design philosophy, from static and periodic devices to reconfigurable and multifunctional circuits.
From Photonic Crystals to PIC Design Methodologies
As designers move from studying photonic crystals in academic settings to developing practical quantum-enhanced circuits, the complexity of the device architectures often increases. PIC design involves assembling a variety of optical components—waveguides, directional couplers, resonators, phase shifters, and detectors—onto a single chip. Unlike photonic crystals, which rely heavily on periodicity and bandgap engineering, PICs often draw inspiration from microwave circuit theory, leveraging well-understood building blocks and carefully controlled refractive index contrasts to guide light along defined paths.
However, these designs do not emerge fully formed from theory. They require extensive modeling to ensure that the chosen waveguide geometries and layouts function as intended. PIC simulations must account for modal analysis, propagation losses, bending losses in curved waveguides, and coupling efficiencies at interfaces. For quantum-enhanced circuits, these considerations become even more stringent because single-photon operations demand very low loss and minimal mode distortion. Achieving the kind of performance needed for quantum photonic devices means that simulation results must be accurate and that their assumptions reflect real fabrication conditions and material properties.
This is where tools like Lumerical and Synopsys come into play. By offering comprehensive simulation platforms, they allow designers to predict device behavior before risking time and resources on fabrication runs. They also help identify parameter sensitivities, explore alternative layouts, and find design compromises between ideal optical performance and practical manufacturing constraints. In essence, while photonic crystals provided the early conceptual triumph of controlling light at the nanoscale, PIC simulation tools ensure that such control can be translated into functioning devices that operate under realistic conditions.
Introduction to Lumerical and Synopsys PIC Simulation Tools
Lumerical and Synopsys have become household names in the integrated photonics community, each offering a suite of simulation modules that cater to different stages of PIC design. Lumerical (now part of Ansys) specializes in photonic device design, offering tools like FDTD (finite-difference time-domain) and MODE for waveguide and device-level simulations, as well as INTERCONNECT for circuit-level analysis. Synopsys, known widely in the semiconductor industry, provides its own set of photonics simulation solutions integrated into a larger ecosystem of electronic-photonic design automation.
At a high level, these tools share a common goal: to allow designers to build virtual models of PIC components and systems, run simulations to predict optical performance, and refine designs iteratively. However, the way these tools achieve their goals can differ, and understanding these differences is vital for learners. Lumerical’s FDTD and MODE solvers excel in analyzing small-scale, device-level phenomena, such as the resonances of a ring resonator or the mode profiles in a photonic crystal waveguide. Synopsys, on the other hand, may emphasize integration with electronic design automation (EDA) tools, offering a pathway to co-simulation of PIC and electronic components for hybrid quantum systems that blend optical and electronic functions.
Educationally, approaching these tools requires patience and a willingness to experiment. The graphical user interfaces can appear complex, and the large number of parameters and solver options might initially overwhelm beginners. Documentation and tutorials provided by the software developers can help, but learners should also be prepared to consult academic literature, join community forums, and engage with colleagues who have prior experience. As with any sophisticated tool, mastery comes not from rote memorization of menu options, but from understanding underlying principles and knowing how to apply them in varied contexts.
Setting Up a PIC Simulation: Fundamental Considerations
Before launching into Lumerical or Synopsys, it is critical to establish a clear set of design goals and constraints. Even a simple component, such as a straight optical waveguide, can be simulated in multiple ways depending on the desired outcomes. For instance, if the objective is to study how waveguide width affects mode confinement, the simulation might involve a parametric sweep over geometrical parameters and careful analysis of the resulting mode profiles. If the goal is to characterize bending losses in a waveguide corner, the simulation might focus on a curved geometry and incorporate material dispersion data.
Quantum-enhanced circuits bring additional complexity, as the simulation must support scenarios like single-photon input states or low-level intensity signals. While neither Lumerical nor Synopsys directly solves the quantum state evolution within PICs in a fully quantum mechanical manner, they can approximate key aspects by treating the photonics hardware classically and ensuring that loss, dispersion, and coupling efficiencies are well understood. This understanding allows designers to later integrate these parameters into a higher-level quantum model that simulates quantum operations on the device.
Throughout the simulation process, users must remain mindful of mesh resolution, computational domain size, and boundary conditions. Electromagnetic solvers rely on discretizing the domain, and too coarse a mesh might yield inaccurate results, while too fine a mesh may push computational requirements beyond practical limits. Similarly, boundary conditions that poorly represent the far-field environment can lead to spurious reflections that distort results. Thus, the simulation setup itself becomes an art of balancing accuracy and resource limitations.
Comparing Photonic Crystal and PIC Simulation Approaches
When analyzing photonic crystals using Lumerical or Synopsys, the focus often centers on band structure calculations, frequency-dependent transmission, and Q-factor evaluations for resonators. FDTD simulations of photonic crystals might involve injecting a broadband pulse into a periodic structure and recording the transmitted and reflected spectra. By identifying frequency bands where transmission is suppressed, one can infer the presence of a photonic bandgap. Although this exercise can be educational, it often remains an academic pursuit rather than a direct path to device realization.
In contrast, PIC simulation methodologies involve more targeted studies. Instead of analyzing full band structures, PIC designers often concentrate on point evaluations of device performance: insertion loss in a coupler, reflectivity from a facet, or resonance wavelengths in a ring resonator. This shift in focus reflects the different design philosophies. PICs are constructed from known building blocks with well-defined functionalities. The objective is to fine-tune these building blocks to meet system-level requirements for quantum-enhanced circuits. The simulation might thus revolve around optimizing a directional coupler’s length and gap size to achieve a certain splitting ratio at a specific wavelength, or ensuring that a mode converter transitions smoothly from a ridge waveguide to a slot waveguide.
Embracing this difference is key to understanding how to best use Lumerical and Synopsys tools. For learners accustomed to photonic crystal simulations, the transition may feel like moving from broad theoretical exercises to more practical and granular optimization tasks. Both approaches have value, but the PIC perspective demands a stronger focus on real-world manufacturability, component interoperability, and system integration.
Addressing Fabrication and Material Constraints in Simulations
Real PICs must be fabricated, and this fabrication step imposes constraints that simulations must acknowledge. Lumerical and Synopsys provide ways to incorporate material dispersion data, surface roughness effects, and other non-idealities that mimic real fabrication conditions. These features remind designers that simulations are not abstract intellectual games, but predictive tools meant to guide decision-making.
For quantum-enhanced circuits, which often require ultra-low loss and strict phase control, simulating realistic conditions becomes especially important. For instance, the absorption coefficient of a particular material might appear negligible at first, but under single-photon-level operation, even minute losses can degrade the circuit’s quantum fidelity. Similarly, waveguide sidewall roughness might introduce scattering losses that are tolerable in classical communication systems but unacceptable for quantum photonic operations.
Incorporating these considerations demands more than just toggling a few options in the simulation software. It requires collaboration with materials scientists, consultation of foundry documentation, and careful interpretation of experimental data. If a foundry specifies a certain process design kit (PDK) with defined tolerances for waveguide width or thickness, the simulation must respect these boundaries. At the same time, designers might use simulation to test the sensitivity of device performance to these tolerances, identifying which parameters are most critical and where manufacturing improvements would yield the greatest gains.
Educating the Next Generation of PIC Designers
As the integrated photonics ecosystem matures, education and training become central to ensuring that the next generation of designers can effectively use tools like Lumerical and Synopsys. University courses and workshops increasingly feature practical sessions where students learn to simulate simple PIC elements. However, teaching simulation is not just about software tutorials. It involves instilling a mindset that recognizes simulation as one step in a larger design cycle that includes theory, fabrication, testing, and iteration.
Encouraging students to start from simpler problems and gradually build complexity can help. For example, beginning with a single straight waveguide simulation allows learners to become familiar with mesh settings, boundary conditions, and mode analysis. Once they gain confidence, they can progress to more complex components, like a Mach-Zehnder interferometer or a ring resonator. Eventually, they can integrate multiple components into a larger quantum-enhanced circuit. This incremental approach fosters deeper understanding and reduces the risk of frustration that might arise from tackling advanced problems too soon.
It is also beneficial to highlight common pitfalls and misunderstandings. Students might, for instance, trust simulation results too blindly, forgetting that all models rely on assumptions and approximations. Encouraging critical thinking and a willingness to validate simulation outputs with experimental data, when available, can mitigate this risk. By incorporating discussions about experimental uncertainties, fabrication challenges, and material property variations, educators can help students become more well-rounded PIC designers.
Organizational and Cultural Factors Shaping PIC Simulation
Effective PIC simulation is not solely a technical matter. Organizational and cultural factors influence how tools like Lumerical and Synopsys are adopted, shared, and improved. In academic institutions, a culture that values open-source tools and reproducibility might encourage code sharing, custom script development, and the publication of simulation parameters for community validation. In contrast, industrial settings often prioritize proprietary workflows, process confidentiality, and integration with other proprietary EDA tools.
These differences affect how newcomers learn to use the software. In academia, one might find abundant free tutorials, user forums, and community-driven documentation, making it easier for students to explore the tools. However, academic users sometimes lack access to the latest foundry-specific PDKs or advanced modules due to licensing costs. Industry professionals might have access to better-integrated workflows and advanced material databases, but fewer opportunities to discuss their work openly or share simulation files publicly.
Navigating these cultural landscapes requires an awareness of the environment in which one operates. Students moving into industry positions might need to adapt to more restrictive policies, while industry engineers who return to academia for advanced research may find the open exchange of ideas refreshing but less structured. Understanding these cultural dynamics is as important as mastering the technical aspects of simulation. After all, PIC design is a collaborative endeavor, and effective communication and expectation management are as crucial as technical prowess.
The Role of Policy, Funding, and Standardization in PIC Simulation
Policy measures and funding frameworks can either accelerate or impede progress in PIC simulation. Large-scale research initiatives, government grants, and consortiums that support integrated photonics development often emphasize the importance of design tools and design automation. Some programs allocate funds for developing foundry-specific PDKs, standardizing component libraries, or creating educational materials that demystify complex simulation processes.
This top-down influence can have a trickle-down effect on learners and practitioners. If policymakers and funding agencies recognize the importance of simulation tools, they might support the integration of Lumerical and Synopsys in academic curricula, fund open-access workshops, or encourage the development of user-friendly interfaces and documentation. Conversely, if policy attention drifts elsewhere, the community might struggle with outdated tools, fragmented resources, and a lack of standardized best practices.
Standardization is another area where policy can have a profound impact. Just as the semiconductor industry benefits from well-defined standards for transistor dimensions and performance metrics, the PIC community could benefit from standardized simulation benchmarks, open databases of material properties, and consensus on how to report losses, coupling efficiencies, and mode conversion ratios. Such standardization would make it easier for learners to trust simulation results, compare different designs, and build upon prior work without revalidating every parameter. While progress is being made, achieving these standards remains a long-term challenge that will require sustained cooperation among academics, industry, and regulatory bodies.
Challenges in Scaling Quantum-Enhanced PICs
Quantum-enhanced circuits push the requirements for PIC design to the extreme. Losses that might be acceptable for classical communication systems become prohibitive at the single-photon level. Imperfections in coupling or phase control that once could be tolerated now directly affect quantum gate fidelity. Ensuring that simulation tools can accurately predict and mitigate these effects is a central challenge.
Another difficulty lies in bridging the gap between classical electromagnetic simulations and the quantum mechanical behavior of the circuits. While Lumerical and Synopsys can predict how light propagates, reflects, and couples in linear and nonlinear materials, they do not inherently simulate quantum states or entanglement. Designers typically must extract classical parameters (such as insertion loss, phase shift, and bandwidth) and feed them into separate quantum simulation frameworks or analytical models. This two-step approach can be cumbersome and prone to misinterpretation. Educational efforts must therefore extend beyond PIC simulation tools, helping learners understand how to connect classical device simulations with quantum information protocols.
A final challenge arises from the need for scalability. Quantum-enhanced circuits often require arrays of identical devices or large networks of interconnected components. Simulating a single device might be feasible, but scaling up to hundreds or thousands of devices is computationally demanding. Learners should be aware that full system simulations might remain out of reach for the foreseeable future, requiring approximation techniques, hierarchy-based modeling, or reduced-order models.
Continuous Improvement Through Experiment-Simulation Feedback Loops
No matter how advanced simulation tools become, experimental validation remains essential. One best practice is to engage in experiment-simulation feedback loops, where a device is simulated, fabricated, tested, and then refined based on discrepancies between simulation and measurement. This iterative process helps identify which assumptions in the simulation are too idealized and where improvements in the material models, boundary conditions, or mesh resolution are needed.
In educational settings, it may be challenging to provide students with hands-on fabrication and testing experiences. Nevertheless, educators can introduce the concept of validation by discussing published studies where authors simulate a device, report expected behavior, and then compare it to experimental data. Through these examples, learners gain an appreciation for the iterative nature of PIC design, the uncertainties in simulation parameters, and the importance of maintaining a critical mindset.
By acknowledging that simulation alone does not guarantee success, learners will be better prepared to navigate a field where designs must stand up to the scrutiny of real-world conditions. This perspective also fosters humility, reminding designers that even the most advanced software cannot replace empirical evidence or the subtle insights gained from working with actual devices.
Embracing Complexity and Encouraging Lifelong Learning
As integrated photonics, quantum-enhanced circuits, and PIC simulation tools continue to evolve, so too must the skill sets of those who engage with them. Lumerical and Synopsys will undoubtedly introduce new features, refine their interfaces, and offer more integration options with other software suites. Meanwhile, emerging platforms may challenge their dominance, bringing fresh approaches to simulation, optimization, and verification.
This dynamic landscape means that learning does not end with completing a single tutorial or mastering a few simulation workflows. Instead, practitioners should embrace the idea of lifelong learning, staying informed about new methodologies, reading the latest research, and revisiting established concepts as technology advances. Such a mindset ensures that users of Lumerical and Synopsys remain adaptable, curious, and prepared to tackle the next generation of photonic challenges.
For educators and mentors, the challenge is to design curricula that instill these values. Courses that blend theoretical foundations with practical simulation exercises, encourage problem-based learning, and showcase real-world case studies can inspire students to become active contributors to the field rather than passive recipients of knowledge. In this way, the educational environment surrounding PIC simulation tools does not merely produce competent engineers and scientists—it shapes innovators who will define the future of integrated photonics.
Conclusion and Call to Action
From photonic crystals to photonic integrated circuits, from pure theoretical constructs to practical quantum-enhanced designs, the journey of integrated photonics is both inspiring and challenging. Lumerical and Synopsys stand as powerful aids along this journey, enabling detailed PIC simulations that guide device optimization, explore design trade-offs, and identify fabrication constraints. Yet these tools, while impressive, are not panaceas. They must be complemented by critical thinking, experimental validation, and a thorough understanding of both the quantum and classical principles at play.
As the field advances, learners must be prepared to engage with complexity, embrace uncertainty, and collaborate across disciplinary boundaries. The challenges are manifold: bridging the gap between simulation and fabrication, ensuring that quantum-enhanced circuits maintain their delicate quantum states, and navigating the organizational and cultural factors that shape how simulation tools are deployed and improved. Addressing these challenges requires a collective effort from policymakers who fund and guide research, educators who develop and refine curricula, engineers who refine simulation workflows, and researchers who push the boundaries of what PICs can achieve.
The call to action is clear. Those entering the field should not shy away from simulation tools due to their complexity, nor should they rely on them without critical scrutiny. Instead, they should view Lumerical, Synopsys, and other PIC simulation platforms as catalysts for learning, experimentation, and innovation. By doing so, the community can accelerate the development of scalable, reliable, and high-performance quantum-enhanced circuits, bringing us closer to realizing the full potential of integrated photonics.
References and Further Reading
Bogaerts, W., & Chrostowski, L. (2018). Photonic integrated circuit design automation: Past, present, and future. IEEE Nanotechnology Magazine, 12(2), 20–29. https://doi.org/10.1109/MNANO.2018.2819409
Foresi, J. S., et al. (1997). Photonic-bandgap microcavities in optical waveguides. Nature, 390(6656), 143–145. https://doi.org/10.1038/36451
Flamini, F., Spagnolo, N., & Sciarrino, F. (2018). Photonic quantum information processing: a review. Reports on Progress in Physics, 82(1), 016001. https://doi.org/10.1088/1361-6633/aad5b2
Knill, E., Laflamme, R., & Milburn, G. J. (2001). A scheme for efficient quantum computation with linear optics. Nature, 409(6816), 46–52. https://doi.org/10.1038/35051009
Lumerical Inc. (2021). Lumerical photonic design tools. https://www.lumerical.com/
Miller, D. A. B. (2017). Attojoule optoelectronics for low-energy information processing and communications. Journal of Lightwave Technology, 35(3), 346–396. https://doi.org/10.1109/JLT.2016.2642860
Nielsen, M. A., & Chuang, I. L. (2010). Quantum Computation and Quantum Information: 10th Anniversary Edition. Cambridge University Press. https://doi.org/10.1017/CBO9780511976667
Politi, A., Matthews, J. C. F., Thompson, M. G., & O’Brien, J. L. (2009). Integrated quantum photonics. IEEE Journal of Selected Topics in Quantum Electronics, 15(6), 1673–1684. https://doi.org/10.1109/JSTQE.2009.2026060
Perez, D., Gasulla, I., & Capmany, J. (2017). Field-programmable photonic arrays. Optics Express, 25(3), 282–294. https://doi.org/10.1364/OE.25.000282
Synopsys Inc. (2021). Photonic solutions. https://www.synopsys.com/optical-solutions.html
Xu, X., et al. (2020). Photonic inverse design: From fundamentals to applications. Advanced Optics and Photonics, 12(3), 828–887. https://doi.org/10.1364/AOP.381966