This article provides a critical evaluation of key material platforms for integrated quantum photonic circuits, emphasizing silicon photonics, III-V semiconductors, and emerging materials that include thin-film lithium niobate, silicon nitride, and two-dimensional crystals. By examining trade-offs related to scalability, fabrication tolerances, propagation losses, nonlinearities, integration complexity, and compatibility with single-photon sources and detectors, the analysis delineates the strengths and weaknesses of each platform. The discussion also contextualizes these materials within organizational, cultural, and policy frameworks that influence production choices and long-term research trajectories. Through a multifaceted perspective that combines technical performance metrics, industrial adaptability, and ecosystem readiness, it offers insights to guide engineers, researchers, and decision-makers in selecting the most suitable material platforms for reliable and scalable quantum photonic solutions.
Dr. Javad Zarbakhsh, Cademix Institute of Technology
Table of Contents
Introduction
The pursuit of integrated quantum photonic circuits encapsulates an aspiration to harness the quantum properties of light in stable, miniature platforms suitable for scalable computation, secure communication, and ultraprecise sensing. As the complexity of quantum devices expands, the material platforms underpinning these systems play a pivotal role in determining device performance, yield, and long-term reliability. The choice of materials is neither trivial nor purely technical. It emerges from a convergence of factors including design constraints, fabrication tolerances, availability of mature processes, organizational cultures within foundries, and the strategic vision set by policymakers and funding agencies. Engineers and researchers find themselves at a crossroads, confronted by a growing array of material platforms—some well-established, others still experimental—each promising unique strengths and presenting distinct challenges.
Within this landscape, silicon photonics stands as a celebrated champion, its popularity fueled by compatibility with existing semiconductor infrastructure, CMOS processes, and the allure of mass production. The lineage of silicon photonics, evolving from decades of microelectronics, offers advantages in scaling, cost efficiency, and design automation. Yet, silicon is far from a universal solution. It lacks direct light emission capabilities, relies on hybrid integration with external gain media, and may impose certain limitations on nonlinear optical properties or the efficient generation of single photons. In response to these constraints, other material platforms—most notably III-V semiconductors—have been developed to incorporate active elements and directly generate and detect photons on-chip.
III-V materials like indium phosphide or gallium arsenide bring their own sets of virtues and complications. They excel in active functionalities, enabling integrated lasers, amplifiers, and quantum-dot-based single-photon emitters. However, their fabrication processes can be more complex and less compatible with silicon-based foundries. The cost structure and yield stability may pose serious scaling barriers, and industrial adoption hinges on a delicate balance between performance metrics and production feasibility.
Emerging materials such as thin-film lithium niobate, silicon nitride, diamond, and two-dimensional crystals like graphene or transition metal dichalcogenides add further layers of complexity. These materials often promise improved nonlinearities, lower propagation losses, or compatibility with advanced quantum states. At the same time, their immaturity in terms of manufacturing readiness, standardization, and process control can restrict their immediate utility. As a result, decision-makers must navigate a labyrinth of trade-offs, guided not only by intrinsic material properties but also by supply chain considerations, workforce skill sets, policy incentives, and a global race to commercialize quantum photonic technologies.
This article undertakes a comparative analysis of these material platforms, exploring their technical attributes while also attending to organizational, cultural, and market dimensions. From silicon photonics to III-V platforms and beyond, the discussion aims to illuminate the criteria that will shape future investments and research directions. The conclusion underscores the need for informed, context-sensitive choices that appreciate not only the physical parameters of materials but also the ecosystems in which they are developed and deployed.
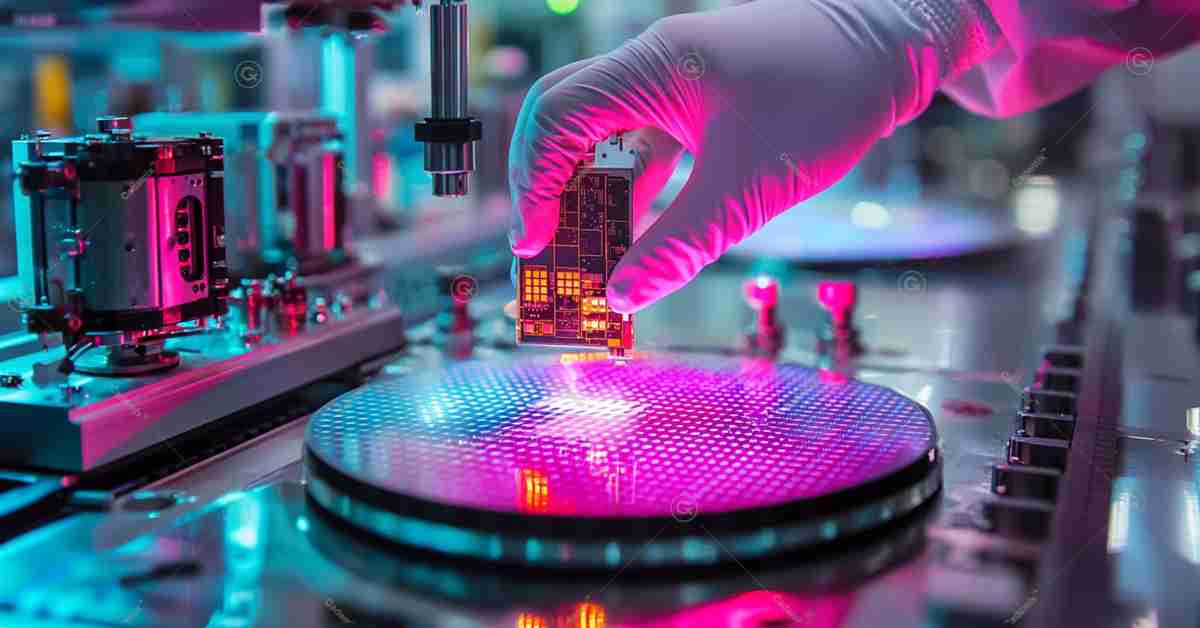
Silicon Photonics: Ubiquity and Limitations
Silicon photonics enjoys a distinguished position in integrated photonics, having emerged from decades of research and industrial progress. Its appeal lies in leveraging existing CMOS infrastructure, enabling wafer-scale production and compatibility with advanced lithographic tools. The stable, low-cost fabrication processes and the possibility of integrating photonics with microelectronics on the same substrate create a compelling narrative for silicon as the go-to platform. In the context of quantum photonics, silicon waveguides have proven adept at implementing linear optical circuits for path-encoded qubits, interference-based logic, and passive structures like directional couplers and phase shifters. The fine feature resolution achievable on silicon chips is a boon for complex quantum circuits requiring precise control over mode profiles and path lengths.
However, silicon’s intrinsic material properties limit its direct application to certain quantum functionalities. Silicon is an indirect-bandgap semiconductor, making it inherently inefficient as a light emitter. Realizing on-chip single-photon sources or lasers necessitates hybrid integration of III-V gain materials, quantum dots, or other light-emitting centers. This approach introduces complexity and may hamper yield, reproducibility, and cost advantages. Furthermore, while silicon offers low propagation losses at telecommunications wavelengths, reducing losses to levels suitable for quantum-grade circuits remains challenging. Residual scattering and absorption can degrade the fidelity of entangled states or the coherence of single photons over extended chip areas.
Nonlinear optical processes, essential for many quantum applications—such as the generation of entangled photon pairs or frequency conversion—are not particularly strong in silicon. Although engineering approaches, including leveraging the Kerr effect or employing engineered dispersion, can mitigate some of these weaknesses, the nonlinear coefficients are still moderate. As quantum circuits evolve to require active manipulation of quantum states through nonlinear processes, silicon’s limitations become apparent. The reliance on sophisticated packaging, the complexity of hybrid bonding, and the stringent cleanliness of fabrication environments amplify organizational and logistical challenges.
Cultural and policy aspects also influence the adoption of silicon photonics for quantum applications. The heavy investment in silicon fabs worldwide and the existence of standardized process design kits (PDKs) encourage its use, but also create inertia. Organizations comfortable with silicon may hesitate to explore alternatives due to sunk costs and established supply chains. Policymakers, seeing silicon as a safe bet, may direct funding toward incremental improvements rather than supporting radical experiments in other materials. Overcoming these tendencies requires visionary leadership and incentives for foundries and researchers to push beyond the comfort zone of silicon.
III-V Semiconductors: Active Functionality and Complex Integration
If silicon photonics represents the backbone of passive quantum circuits, III-V semiconductors may be considered the heart and soul of active functionality. Materials such as indium phosphide or gallium arsenide offer direct-bandgap emission, enabling integrated lasers, modulators, and detectors without the need for complex hybridization. This direct emission capacity simplifies the generation of single photons, a core requirement for many quantum information protocols. The integration of quantum dots, for instance, allows the generation of indistinguishable single photons on demand, a critical capability for photonic quantum computing and secure communication schemes.
III-V platforms also boast stronger nonlinearities compared to silicon, facilitating efficient parametric down-conversion or sum-frequency generation. Such nonlinear processes enable the production of entangled photons and frequency conversion between different spectral bands, vital for interfacing disparate quantum systems. By incorporating quantum wells, wires, or dots, III-V materials can produce quantum emitters with well-defined properties, pushing integrated photonic chips beyond mere passive circuitry and into the realm of fully functional quantum devices.
Nevertheless, III-V integration comes with considerable challenges. Fabrication processes for III-V materials are less standardized than CMOS lines, making wafer-scale production expensive and less reproducible. Yields can suffer, and process variations between foundries can complicate multi-sourced supply chains. The thermal and mechanical stability of III-V materials may be weaker than silicon’s, raising reliability questions under continuous operation or cryogenic conditions. Packaging solutions, which must ensure coupling to external fibers or waveguides while preserving quantum coherence, add layers of complexity that few organizations have fully mastered.
From an organizational standpoint, shifting to III-V materials demands new skill sets, design methodologies, and metrology techniques. Research institutions might lead the charge, but industrial foundries may resist retrofitting their lines or adopting entirely new toolsets. The cultural mindset that prizes silicon’s familiarity can slow adoption of III-V solutions. Without policy interventions encouraging diversification or strategic partnerships that reduce cost barriers, III-V materials might remain niche solutions favored by specialized research groups or high-end applications rather than a mainstream platform.
Emerging Materials: Opportunities and Uncertainties
Beyond silicon and III-V semiconductors, a blossoming ecosystem of emerging materials promises breakthroughs in integrated quantum photonics. Thin-film lithium niobate, for example, has shown exceptional promise due to its large electro-optic coefficient, enabling fast and efficient modulation of photons on chip. This capability is pivotal for advanced quantum gates and reconfigurable circuits. Similarly, silicon nitride waveguides, with their ultralow losses, pave the way for propagation of quantum states over larger chip areas without significant decoherence. When combined with techniques for coupling to quantum emitters, these platforms can yield integrated devices with unprecedented coherence times and stability.
Diamond, with its nitrogen-vacancy centers, offers stable single-photon emission and long spin coherence times, bridging the gap between photonics and quantum memories. Two-dimensional materials like graphene or transition metal dichalcogenides hold promise for ultrafast modulation, nonlinear phenomena, and integration with unconventional photonic architectures. However, these materials remain at varying levels of technological maturity. While their intrinsic properties may surpass established platforms in certain metrics, the reproducibility, fabrication scaling, and long-term reliability remain largely unproven.
Emerging materials also face cultural and organizational hurdles. Investors and policymakers often prefer incremental improvements in known platforms rather than backing unproven materials. As a result, research on emerging materials may struggle to secure stable funding. The organizational knowledge required to handle these materials—from nanofabrication expertise to specialized metrology—does not transfer seamlessly from silicon or III-V processes. Companies risk lengthy development cycles and uncertain returns, discouraging large-scale adoption until clear market advantages are demonstrated.
Policy frameworks and global research agendas can influence the trajectory of emerging materials. Targeted funding calls that support exploratory research, testbeds that foster collaborative experimentation, and open-access design libraries can all accelerate the maturity of these platforms. Without such support, emerging materials risk falling into a trap: remaining perpetually “on the horizon” while the industry continues to refine incumbent solutions.
Performance Metrics, Fabrication Challenges, and System Integration
Comparing these material platforms requires a systematic look at performance metrics and the underlying fabrication challenges. Key metrics include propagation losses, mode confinement, nonlinear coefficients, thermal stability, and compatibility with single-photon sources and detectors. Silicon excels in propagation losses at telecom wavelengths, achieving waveguide attenuation as low as a few dB/m, but its nonlinearities and emission capabilities lag behind. III-V materials excel in active functionalities, providing integrated gain and strong nonlinearities, yet their losses and yield issues can detract from their appeal.
Emerging materials may offer the best of both worlds—high nonlinearities and low losses—but face scaling challenges. Maintaining uniform thickness in lithium niobate films, controlling defect densities in diamond, or reliably transferring 2D materials onto substrates all represent formidable engineering hurdles. The complexity of these processes may require specialized equipment, stringent environment controls, and iterative optimization cycles before achieving acceptable yields.
System integration also matters. A quantum photonic chip rarely functions in isolation. It must interface with external sources, detectors, and sometimes cryogenic environments. Packaging solutions that provide fiber-to-chip coupling with low insertion loss, stable mechanical alignment, and minimal back reflections are nontrivial. The chosen material platform influences packaging complexity. Silicon photonics benefits from a wide range of standardized packaging tools, while III-V or emerging materials may require custom approaches. Organizational cultures that encourage supply chain collaborations, shared test facilities, and standardization efforts can alleviate these integration difficulties.
At the cultural level, engineers and scientists must learn to think holistically, appreciating how material choices influence downstream assembly steps and overall system performance. Education and workforce development strategies that emphasize interdisciplinary training can help break down silos. Policymakers might support initiatives that develop standardized packaging protocols or shared foundry services, reducing the learning curve and encouraging cross-pollination between material platforms.
Conclusion and Call to Action
The comparative landscape of material platforms for integrated quantum photonic circuits reveals a complex tapestry of trade-offs. Silicon photonics, with its established infrastructure and compatibility with CMOS processes, provides a strong foundation for passive devices and large-scale integration, yet struggles in areas requiring on-chip light emission or strong nonlinear processes. III-V semiconductors shine in active functionalities, enabling integrated sources and detectors, but incur greater fabrication complexity and cost. Emerging materials introduce tantalizing possibilities for enhanced nonlinearities, ultralow losses, and novel functionalities, yet remain largely unproven at industrial scales and risk marginalization without strategic support.
These choices unfold within a broader context shaped by market incentives, policy directives, organizational cultures, and educational ecosystems. Policymakers who prioritize certain material platforms through funding, industry consortia that develop and share best practices, and research institutions that invest in interdisciplinary training all exert influence on which platforms gain traction. Without carefully orchestrated efforts to align technical capabilities with practical manufacturability and market relevance, the field could stagnate in incremental improvements rather than achieving transformative breakthroughs.
Addressing these challenges requires a collective call to action. Researchers must refine understanding of material properties, pushing for new characterization methods and simulation tools that guide platform selection. Foundries and companies should collaborate to develop standardized process design kits and packaging protocols for a broader range of materials. Policymakers can incentivize exploration of emerging platforms, encouraging risk-taking and technology transfer to reduce barriers to industrial adoption. Educational institutions, in turn, can train a new generation of engineers and scientists adept at navigating multiple material platforms, comfortable with both technical details and the strategic contexts shaping their application.
As quantum photonic circuits inch closer to commercialization and large-scale deployment, material choices will matter more than ever. The strategic selection of platforms can spell the difference between devices that remain laboratory curiosities and those that power the next generation of quantum networks, sensors, and computers. By acknowledging these complexities and acting deliberately to support a diverse ecosystem of materials, stakeholders can pave the way for resilient, high-performance quantum photonic technologies that unlock the promise of this new quantum era.
References and Further Reading
Bogaerts, W., & Chrostowski, L. (2018). Photonic integrated circuit design automation: past, present, and future. IEEE Nanotechnology Magazine, 12(2), 20–29.
https://doi.org/10.1109/MNANO.2018.2819409
Flamini, F., Spagnolo, N., & Sciarrino, F. (2018). Photonic quantum information processing: a review. Reports on Progress in Physics, 82(1), 016001.
https://doi.org/10.1088/1361-6633/aad5b2
Nielsen, M. A., & Chuang, I. L. (2010). Quantum Computation and Quantum Information: 10th Anniversary Edition. Cambridge University Press.
https://doi.org/10.1017/CBO9780511976667
Perez, D., Gasulla, I., & Capmany, J. (2017). Field-programmable photonic arrays. Optics Express, 25(3), 282–294.
https://doi.org/10.1364/OE.25.000282
Politi, A., Matthews, J. C. F., Thompson, M. G., & O’Brien, J. L. (2009). Integrated quantum photonics. IEEE Journal of Selected Topics in Quantum Electronics, 15(6), 1673–1684.
https://doi.org/10.1109/JSTQE.2009.2026060
Soref, R. (2010). The past, present, and future of silicon photonics. IEEE Journal of Selected Topics in Quantum Electronics, 16(1), 167–176.
https://doi.org/10.1109/JSTQE.2009.2035196
Wang, J., et al. (2018). Multidimensional quantum entanglement with large-scale integrated optics. Science, 360(6386), 285–291.
https://doi.org/10.1126/science.aar7053
Xu, X., et al. (2020). Photonic inverse design: from fundamentals to applications. Advanced Optics and Photonics, 12(3), 828–887.
https://doi.org/10.1364/AOP.381966
Zhang, H., et al. (2021). Integrated quantum photonics: current status and future prospects. APL Photonics, 6(7), 071101.
https://doi.org/10.1063/5.0045024
European Quantum Flagship. (2021). Strategic Research Agenda for Quantum Technologies.
https://qt.eu/roadmap