The integration of Photovoltaic Modules into building facades presents a significant opportunity for enhancing energy efficiency in urban environments. This study explores the design considerations, architectural flexibility, and efficiency potential of facade-integrated PV systems, with a focus on applications in Europe, particularly Germany and Austria. The analysis addresses the technical aspects of different PV technologies, emphasizing their impact on energy generation, building aesthetics, and structural integrity.
Regulatory frameworks and economic factors, including costs, incentives, and return on investment, are also examined to provide a comprehensive overview of the feasibility and benefits of such systems. Through case studies of successful implementations, the article highlights best practices and emerging trends, offering valuable insights for architects, engineers, and policymakers aiming to incorporate sustainable energy solutions into urban design. The findings underscore the role of facade-integrated PV in achieving sustainability goals, while also showcasing the author’s expertise in this rapidly evolving field. By Alireza Alidadi, Cademix Institute of Technology
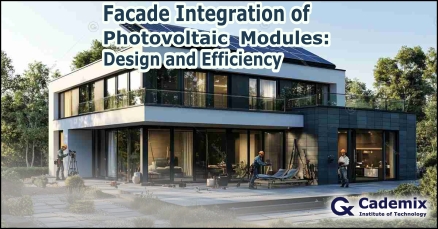
Introduction
As the global community increasingly turns its attention to sustainable energy solutions, the integration of photovoltaic (PV) modules into building facades emerges as a crucial innovation. Urban environments, with their dense concentration of buildings and limited space for traditional solar installations, present unique challenges and opportunities for energy generation. Facade-integrated photovoltaic (BIPV) systems offer a promising solution that not only meets energy needs but also aligns with modern architectural aesthetics.
Photovoltaic technology, which converts sunlight directly into electricity, has traditionally been deployed on rooftops. However, as cities grow vertically and available roof space diminishes, the need to utilize other building surfaces becomes apparent. Facades, which constitute a significant portion of a building’s surface area, present an untapped resource for energy generation. By integrating PV modules into these surfaces, buildings can produce clean energy while maintaining or even enhancing their visual appeal.
The significance of facade-integrated PV systems extends beyond their technical capabilities. In Europe, particularly in countries like Germany and Austria, where sustainability is deeply embedded in public policy, the integration of renewable energy sources into urban infrastructure is not just encouraged but often mandated. The European Union’s commitment to reducing carbon emissions and increasing the share of renewable energy in its energy mix provides a robust regulatory framework that supports the adoption of BIPV systems.
Architectural flexibility is another key advantage of facade-integrated PV systems. Unlike conventional solar panels, which are typically installed on rooftops with a one-size-fits-all approach, facade-integrated modules can be customized to match the design and aesthetic requirements of different buildings. This flexibility allows architects and engineers to create visually appealing buildings that contribute to the energy grid without compromising on design integrity.
The efficiency of facade-integrated PV systems, however, is influenced by several factors, including the orientation and angle of the building, the type of PV technology used, and the environmental conditions of the urban setting. In this context, understanding the relationship between design choices and energy output becomes essential. Urban planners and engineers must consider these factors when integrating PV systems into building facades to optimize energy generation.
This article aims to provide a comprehensive overview of facade-integrated PV systems, focusing on design considerations, efficiency, and the economic and regulatory landscape in Europe. By examining case studies from Germany and Austria, it will highlight best practices and emerging trends that could shape the future of urban energy generation. The goal is to offer insights that are valuable not only to architects and engineers but also to policymakers and developers who are involved in the sustainable development of urban spaces.
Ultimately, the integration of PV modules into building facades represents a significant step forward in the quest for sustainable urban environments. As the technology continues to evolve, it holds the potential to transform the way we think about building design and energy generation, making our cities not only more energy-efficient but also more resilient and sustainable.
Section 1: Overview of Photovoltaic Technology
1.1 Basics of Photovoltaic Cells
Photovoltaic (PV) technology, at its core, is about harnessing sunlight and converting it into electricity. This process relies on the photovoltaic effect, a phenomenon where certain materials generate an electric current when exposed to sunlight. The basic unit of this technology is the photovoltaic cell, which is typically made from semiconductor materials such as silicon. These cells are the building blocks of PV modules, which are commonly referred to as solar panels.
Photovoltaic cells are primarily classified into three main types based on the materials and manufacturing processes used: monocrystalline, polycrystalline, and thin-film.
- Monocrystalline PV cells are made from a single continuous crystal structure, typically silicon. They are known for their high efficiency and durability, making them a popular choice for many solar installations. However, they are also more expensive due to the complexity of the manufacturing process.
- Polycrystalline PV cells are composed of multiple silicon crystals. While they are less efficient than monocrystalline cells, they are also less expensive, making them a cost-effective option for many applications.
- Thin-film PV cells are made by depositing one or more thin layers of photovoltaic material onto a substrate, such as glass or metal. These cells are flexible and lightweight, making them suitable for a variety of applications, including integration into building materials. However, they tend to have lower efficiency compared to crystalline silicon cells.
The choice of PV cell type for facade integration depends on various factors, including the desired balance between efficiency, cost, and aesthetic considerations. Understanding these basics is crucial as we explore the more advanced aspects of integrating PV technology into building facades.
1.2 Current Trends in Photovoltaic Technology
The field of photovoltaic technology is rapidly evolving, driven by the need for more efficient, cost-effective, and aesthetically pleasing solutions. In recent years, there have been significant advancements in PV materials, manufacturing processes, and system designs, all of which have implications for their integration into building facades.
One notable trend is the development of bifacial PV modules, which can capture sunlight from both sides, thereby increasing energy yield. When integrated into facades, these modules can harness reflected light from the surrounding environment, which is particularly useful in urban areas where buildings are often closely spaced. Bifacial modules are becoming increasingly popular in Europe, where maximizing energy generation from limited surface areas is a key concern.
Another trend is the advancement of perovskite-based PV cells, which promise higher efficiencies and lower production costs compared to traditional silicon-based cells. Perovskites have the added advantage of being more versatile in terms of application, including the potential for integration into transparent or semi-transparent modules. This opens up new possibilities for facade integration, allowing buildings to generate electricity without sacrificing natural light or architectural design.
Building-integrated photovoltaics (BIPV) are also gaining traction, particularly in Europe. Unlike traditional solar panels, which are added to buildings as an external feature, BIPV systems are integrated directly into the building materials, such as windows, facades, or roofing. This integration not only saves space but also reduces the need for additional construction materials, aligning with the principles of sustainable design.
As urban areas continue to expand and the demand for renewable energy grows, these trends are shaping the future of PV technology. The challenge lies in effectively integrating these innovations into building designs in a way that maximizes their potential while also meeting aesthetic and functional requirements.
1.3 Why use Integration of Photovoltaic Modules into Facades?
Traditionally, PV systems have been installed on rooftops, where they can capture the maximum amount of sunlight without significant obstructions. However, as cities become more densely populated and buildings grow taller, the availability of rooftop space diminishes. This limitation has led to a growing interest in integrating PV modules into building facades.
Facade integration offers several advantages over traditional rooftop installations. Firstly, space utilization is significantly improved. In urban environments, where land is scarce and expensive, utilizing vertical surfaces for energy generation makes practical sense. By integrating PV modules into facades, buildings can generate electricity across a much larger surface area than would be possible with rooftop systems alone.
Secondly, facade-integrated PV systems can contribute to energy efficiency in ways that go beyond electricity generation. For instance, PV modules can also serve as a shading device, reducing the amount of direct sunlight that enters the building. This can help lower cooling demands and improve the overall thermal performance of the building.
Moreover, the aesthetic potential of facade-integrated PV systems is a compelling reason for their adoption. Unlike traditional solar panels, which are often viewed as an afterthought or a purely functional addition, facade-integrated PV modules can be designed to complement or even enhance the building’s appearance. This opens up new possibilities for architects and designers to create buildings that are not only energy-efficient but also visually striking.
Finally, integrating PV into facades aligns with the broader goals of sustainable urban development. As cities aim to reduce their carbon footprint and meet renewable energy targets, BIPV systems offer a practical solution that can be implemented on a large scale. In Europe, where there is strong regulatory support for renewable energy, facade-integrated PV systems are becoming an increasingly attractive option for new constructions and renovations alike.
In conclusion, the integration of PV modules into building facades represents a strategic response to the challenges of urban energy generation. By leveraging advancements in PV technology and considering the unique characteristics of urban environments, facade-integrated PV systems have the potential to play a significant role in the transition to sustainable energy. This section has provided an overview of the key concepts and trends in PV technology, setting the stage for a deeper exploration of the design, efficiency, and economic considerations that will be discussed in the following sections.
Section 2: Design Considerations for Facade-Integrated PV Systems
2.1 Architectural Design Integration
The integration of photovoltaic (PV) modules into building facades is not just a technical challenge; it is also a significant architectural opportunity. Facade-integrated PV systems can be designed to blend seamlessly with a building’s aesthetics or even enhance its visual appeal. This section explores how architectural design can harmonize with energy generation, focusing on the strategies and techniques that allow PV modules to become an integral part of building design.
Customization and Flexibility in Design:
One of the most compelling aspects of facade-integrated PV systems is their ability to be customized to meet specific architectural needs. Unlike conventional solar panels, which are often installed as uniform arrays on rooftops, facade-integrated PV modules can be designed in various shapes, sizes, and colors. This flexibility allows architects to incorporate PV technology into a wide range of building styles, from modern glass facades to traditional stone or brick exteriors.
For example, PV modules can be manufactured in different colors to match or complement the building’s exterior. This can be particularly important in historic or culturally significant areas, where maintaining the visual integrity of the surroundings is crucial. Furthermore, the use of semi-transparent or even fully transparent PV modules allows for integration into windows or curtain walls, enabling buildings to generate electricity while still allowing natural light to enter.
Case Studies: Germany and Austria
Germany and Austria have been at the forefront of adopting facade-integrated PV systems, driven by strong regulatory support and a commitment to sustainable architecture. For instance, the “Solar Decathlon Europe” competition, which has seen participation from German and Austrian universities, has showcased innovative designs where PV modules are seamlessly integrated into building facades. These designs not only meet energy efficiency standards but also push the boundaries of architectural creativity.
A notable example is the BIQ House in Hamburg, Germany, which features a bio-adaptive facade that includes PV panels. This building demonstrates how PV integration can be combined with other sustainable technologies to create a highly efficient and aesthetically pleasing structure. In Austria, the PLUS-Energy Office Building in Vienna is another example where facade-integrated PV systems have been used to achieve a net-positive energy balance, all while maintaining a modern architectural aesthetic.
Design Challenges and Solutions
Despite the opportunities, integrating PV modules into building facades presents several challenges. One of the primary concerns is maintaining the balance between energy efficiency and aesthetic appeal. Architects and engineers must work closely to ensure that the placement and design of the PV modules do not detract from the building’s overall appearance. This often involves careful consideration of the module’s color, texture, and reflectivity.
Another challenge is ensuring that the structural integrity of the building is not compromised. Facade-integrated PV modules add weight and require secure mounting systems. Additionally, the facade must be designed to accommodate the electrical connections and inverters required to convert the generated DC power into usable AC electricity. Innovative solutions, such as frameless PV modules or modules that are integrated into the building’s glass facade, can help address these challenges while maintaining a sleek appearance.
2.2 Structural and Engineering Considerations
Integrating PV modules into building facades involves more than just aesthetic considerations; it also requires careful attention to structural and engineering details. The additional weight, electrical infrastructure, and potential impact on building performance all need to be taken into account to ensure the successful implementation of facade-integrated PV systems.
Load-Bearing Capacity and Structural Implications
One of the primary engineering challenges of facade-integrated PV systems is the additional load they impose on the building structure. PV modules, along with their mounting systems, add weight to the facade, which can affect the building’s load-bearing capacity. Engineers must ensure that the building can support this additional load without compromising safety or structural integrity.
This consideration is particularly important in retrofitting existing buildings, where the original structure may not have been designed to accommodate the added weight of PV modules. In such cases, reinforcing the facade or selecting lightweight PV technologies, such as thin-film modules, may be necessary to mitigate structural concerns.
Impact on Building Insulation and Thermal Performance
Facade-integrated PV systems can also influence the building’s thermal performance. PV modules can act as an additional layer of insulation, reducing heat loss in winter and heat gain in summer. However, this can also affect the building’s natural ventilation, requiring careful planning to ensure that the building remains comfortable and energy-efficient.
In some cases, the integration of PV modules into the facade can lead to overheating of the modules, which reduces their efficiency. To address this, engineers may incorporate ventilation gaps or cooling systems behind the PV panels to dissipate heat. Alternatively, the use of advanced materials, such as low-emissivity coatings on the glass, can help reduce the thermal load without sacrificing energy generation.
Electrical Infrastructure and Integration
The electrical infrastructure required for facade-integrated PV systems is another critical consideration. This includes the placement of inverters, wiring, and junction boxes, all of which must be integrated into the building’s design without compromising aesthetics or functionality. In urban settings, where space is often limited, this can be a challenging task.
To streamline the integration, some buildings use centralized inverter systems, where the DC power generated by the PV modules is routed to a central location for conversion to AC power. Alternatively, micro-inverters or power optimizers can be installed at the module level, which can improve system efficiency and simplify wiring but may increase the overall cost.
Case Studies: Engineering Solutions
Successful facade-integrated PV projects in Germany and Austria have demonstrated various engineering solutions to these challenges. For example, the Solaire Building in Berlin integrates PV modules into its facade while using advanced lightweight materials to minimize the additional load on the structure. This approach has allowed the building to achieve a high level of energy efficiency without compromising its structural integrity.
Similarly, in Vienna, the Tech Gate Vienna building uses semi-transparent PV modules integrated into its curtain wall, providing both electricity generation and enhanced insulation. The building’s design includes ventilation gaps behind the PV panels to manage heat dissipation, ensuring that the modules operate at optimal efficiency.
2.3 Aesthetic and Functional Balance
Achieving a balance between aesthetic appeal and functional efficiency is perhaps the most challenging aspect of designing facade-integrated PV systems. The goal is to create buildings that not only meet energy generation targets but also contribute to the architectural landscape.
Strategies for Aesthetic Integration
There are several strategies that architects and designers can use to aesthetically integrate PV modules into building facades. One approach is to use PV modules as design elements, incorporating them into patterns or motifs that enhance the building’s appearance. This can be particularly effective in modern or avant-garde architectural styles, where unconventional materials and designs are often celebrated.
Another strategy is to use color-matching or custom-colored PV modules that blend with the building’s exterior. This approach allows the PV system to be nearly invisible, maintaining the building’s original design intent while still providing energy generation. In historic buildings, where maintaining the original appearance is crucial, this strategy can be particularly effective.
Maximizing Functional Efficiency
While aesthetics are important, the primary function of facade-integrated PV systems is to generate electricity. Therefore, maximizing functional efficiency remains a key consideration. This involves optimizing the orientation and tilt of the PV modules, selecting the most appropriate PV technology for the specific site conditions, and ensuring that shading from adjacent buildings or structures is minimized.
In urban environments, where shading is often unavoidable, the use of bifacial PV modules can help increase energy yield by capturing reflected light from the surrounding area. Additionally, integrating PV modules into high-visibility areas, such as the upper floors of tall buildings, can help maximize exposure to sunlight and improve overall system efficiency.
Case Studies: Balancing Aesthetics and Efficiency
The combination of aesthetic appeal and functional efficiency is exemplified in several buildings in Germany and Austria. The KfW Westarkade building in Frankfurt, for example, features a facade-integrated PV system that is both visually striking and highly efficient. The building’s designers used a combination of custom-colored PV modules and advanced engineering techniques to create a facade that generates electricity while enhancing the building’s overall appearance.
In Austria, the Raiffeisen Tower in Vienna is another example where aesthetics and efficiency have been successfully balanced. The building’s facade integrates PV modules into a dynamic, curvilinear design that not only generates electricity but also serves as a key element of the building’s architectural identity. This project demonstrates how PV integration can be both functional and visually impactful, contributing to the building’s sustainability goals while also making a statement in the urban landscape.
Conclusion of Section 2
Designing facade-integrated PV systems requires a careful balance of architectural aesthetics, structural integrity, and functional efficiency. By considering these factors in the design process, architects and engineers can create buildings that are not only energy-efficient but also visually appealing and structurally sound. The examples from Germany and Austria highlight how innovative design solutions can overcome the challenges of facade integration, setting a standard for future projects in urban energy generation. In the following sections, we will explore the efficiency and performance of these systems, along with the economic and regulatory considerations that influence their adoption.
Section 3: Efficiency and Performance of Facade-Integrated PV Systems
3.1 Factors Affecting Efficiency
The efficiency of facade-integrated photovoltaic (PV) systems is influenced by a multitude of factors, each of which can significantly impact the overall energy output of the system. Understanding these factors is crucial for optimizing the performance of facade-integrated PV systems in urban environments, where space is limited and maximizing energy generation is essential.
Orientation and Angle of the Facade
One of the most critical factors affecting the efficiency of facade-integrated PV systems is the orientation and angle of the building facade. Unlike rooftop PV systems, which can be oriented and tilted to capture the maximum amount of sunlight, facade-integrated systems are constrained by the fixed orientation of the building walls.
In the Northern Hemisphere, facades facing south generally receive the most sunlight throughout the day, making them the most suitable for PV integration. However, in dense urban environments, even south-facing facades may be shaded by nearby buildings, reducing their potential energy output. East- and west-facing facades can also contribute to energy generation, particularly in the morning and late afternoon, but their overall efficiency will be lower than that of a south-facing system.
The angle of the facade relative to the sun’s path also plays a crucial role. Vertical facades receive less direct sunlight than tilted surfaces, which can reduce the efficiency of the PV modules. To mitigate this, some designs incorporate angled PV modules or use building elements like overhangs or louvers to optimize the angle of sunlight striking the modules. Advanced simulation tools can be used during the design phase to model the impact of orientation and angle on energy generation, helping architects and engineers make informed decisions.
Type of Photovoltaic Technology
The type of PV technology used in facade-integrated systems also has a significant impact on efficiency. As discussed in Section 1, different PV technologies, such as monocrystalline, polycrystalline, and thin-film, have varying efficiencies and are suited to different applications.
Monocrystalline PV modules, with their higher efficiency rates, are often preferred for facade integration in space-constrained urban environments. However, their higher cost may not always be justified, particularly in situations where large surface areas are available, and lower-cost polycrystalline or thin-film modules may be more economical.
Thin-film PV modules, while less efficient, offer advantages in terms of flexibility and aesthetic integration. They can be applied directly to glass surfaces, making them suitable for integration into windows or curtain walls. Additionally, thin-film modules perform better in low-light conditions and at non-optimal angles, which can partially offset their lower efficiency in certain applications.
Shading and Urban Environment
Shading is a significant concern for facade-integrated PV systems, particularly in urban environments where buildings are often closely spaced. Even partial shading of PV modules can dramatically reduce their efficiency due to the way solar cells are connected within a module. When one part of a module is shaded, it can act as a bottleneck, reducing the output of the entire module or even the entire string of modules.
To mitigate the impact of shading, careful site analysis and planning are essential. Techniques such as using micro-inverters or power optimizers, which allow individual modules to operate independently, can help minimize the loss of efficiency due to shading. Additionally, bifacial PV modules, which can capture sunlight from both the front and back, are particularly effective in urban environments where reflected light from adjacent buildings and surfaces can contribute to energy generation.
Environmental Factors
Environmental factors such as temperature, humidity, and pollution can also affect the efficiency of facade-integrated PV systems. High temperatures can reduce the efficiency of PV modules, as the electrical output of most solar cells decreases with increasing temperature. In urban areas, the heat island effect—where urban temperatures are higher than surrounding rural areas—can exacerbate this issue.
To counteract temperature-related efficiency losses, some facade-integrated PV systems incorporate passive cooling techniques, such as ventilation gaps behind the modules or the use of thermally conductive materials. These approaches help dissipate heat and maintain the modules at an optimal operating temperature.
Airborne pollutants and dust can also accumulate on the surface of PV modules, reducing their ability to capture sunlight. Regular cleaning and maintenance are essential to ensure that facade-integrated PV systems operate at peak efficiency. In some cases, self-cleaning coatings or materials that resist dirt accumulation may be used to reduce the maintenance burden.
3.2 Energy Generation Potential
Facade-integrated PV systems have significant energy generation potential, particularly in urban environments where traditional rooftop installations may be limited. However, the actual energy output of these systems depends on several factors, including the ones discussed above.
Quantitative Analysis of Energy Generation
The energy generation potential of facade-integrated PV systems can vary widely depending on the building’s location, orientation, and the type of PV technology used. For example, a well-designed facade-integrated PV system on a south-facing building in a city like Munich, Germany, could generate approximately 50-70% of the energy that a similarly sized rooftop system might produce. This percentage may be lower for east- or west-facing facades but can still represent a significant contribution to the building’s overall energy needs.
In dense urban environments, where space is at a premium, the ability to generate even a portion of a building’s energy requirements from its facade can have a substantial impact on reducing reliance on external energy sources. Additionally, the integration of PV modules into large buildings, such as office towers or residential complexes, can result in significant cumulative energy generation, particularly when combined with energy-saving measures such as high-efficiency glazing and insulation.
Case Studies from Germany and Austria
Several case studies from Germany and Austria illustrate the energy generation potential of facade-integrated PV systems. For example, the SOLTAG building in Copenhagen, Denmark (close to Germany), is a model of energy-efficient design, incorporating PV modules into its south-facing facade. The building generates enough electricity to cover a significant portion of its energy needs, demonstrating the viability of facade-integrated PV systems in northern European climates.
In Austria, the Solar City in Linz is another example where facade-integrated PV systems have been successfully implemented. The city’s public buildings feature PV modules integrated into their facades, contributing to the city’s goal of becoming energy self-sufficient. The project demonstrates how large-scale deployment of facade-integrated PV systems can contribute to urban sustainability goals.
Performance Monitoring and Maintenance
To ensure that facade-integrated PV systems continue to operate at their maximum potential, ongoing performance monitoring and maintenance are essential. Monitoring systems can track the energy output of individual modules or arrays, providing real-time data that can be used to identify issues such as shading, dirt accumulation, or equipment failure.
Regular maintenance, including cleaning and inspections, is necessary to keep the system operating efficiently. In some cases, advanced monitoring systems can be integrated with automated cleaning solutions, reducing the need for manual intervention and ensuring that the modules remain clean and free of debris.
3.3 Performance Monitoring and Maintenance
Effective performance monitoring and maintenance are critical to the long-term success of facade-integrated PV systems. Unlike traditional rooftop systems, where access for maintenance is relatively straightforward, facade-integrated systems may present unique challenges due to their vertical orientation and integration into the building envelope.
Monitoring Systems
Performance monitoring systems are an essential component of facade-integrated PV installations. These systems typically include sensors and data loggers that track the energy output of the PV modules, as well as environmental conditions such as temperature and sunlight intensity. The data collected can be analyzed to assess the performance of the system and identify any issues that may be affecting efficiency.
Advanced monitoring systems can provide detailed insights into the performance of individual modules or arrays, allowing for early detection of problems such as shading, dirt accumulation, or electrical faults. In urban environments, where shading patterns can change throughout the day due to the movement of the sun and the presence of nearby buildings, real-time monitoring is particularly valuable.
In addition to energy output, monitoring systems can also track the temperature of the PV modules, providing data that can be used to optimize cooling strategies. For example, if a module is found to be operating at a higher-than-expected temperature, steps can be taken to improve ventilation or reduce heat buildup, thereby enhancing efficiency.
Maintenance Considerations
Maintenance of facade-integrated PV systems is essential to ensure long-term performance and efficiency. The vertical orientation of these systems means that dirt, dust, and pollutants can accumulate on the surface of the modules, reducing their ability to capture sunlight. Regular cleaning is necessary to remove these contaminants and maintain the system’s energy output.
In some cases, self-cleaning coatings or materials that resist dirt accumulation can be used to reduce the need for manual cleaning. These coatings typically rely on hydrophobic or photocatalytic properties to prevent dirt from adhering to the surface of the modules or to break down organic materials when exposed to sunlight.
Access for maintenance can be a challenge with facade-integrated PV systems, particularly on tall buildings or complex facades. To address this, maintenance plans should be developed during the design phase, taking into account the specific characteristics of the building and the PV system. This may involve the installation of dedicated access points, such as catwalks or scaffolding, or the use of specialized equipment, such as robotic cleaners or drones, to perform maintenance tasks.
Best Practices in Monitoring and Maintenance
Best practices in monitoring and maintenance can significantly extend the lifespan of facade-integrated PV systems and ensure that they continue to operate at peak efficiency. These practices include:
- Regular Inspections: Scheduled inspections should be conducted to assess the physical condition of the PV modules, mounting systems, and electrical connections. Any issues should be addressed promptly to prevent further damage or performance degradation.
- Data Analysis: The data collected by monitoring systems should be regularly analyzed to identify trends or anomalies that may indicate a problem. This proactive approach can help prevent minor issues from becoming major failures.
- Cleaning and Maintenance Schedule: A regular cleaning schedule should be established based on the specific environmental conditions of the site. In areas with high levels of pollution or dust, more frequent cleaning may be necessary.
- Use of Technology: Advanced technologies, such as drones or robotic cleaners, can be used to perform inspections and maintenance on difficult-to-reach areas. These technologies can reduce the need for manual labor and improve the safety and efficiency of maintenance operations.
Conclusion of Section 3
The efficiency and performance of facade-integrated PV systems are influenced by a complex interplay of factors, including orientation, technology type, shading, and environmental conditions. By carefully considering these factors during the design phase and implementing effective monitoring and maintenance practices, architects and engineers can optimize the energy generation potential of these systems. The case studies from Germany and Austria highlight the significant contributions that facade-integrated PV systems can make to urban sustainability goals, demonstrating the viability of this technology in real-world applications. The following sections will explore the economic and regulatory considerations that further influence the adoption and success of facade-integrated PV systems.
Section 4: Regulatory and Economic Considerations
4.1 Regulatory Framework in Europe
The integration of photovoltaic (PV) modules into building facades is not only a technical and design challenge but also a matter of navigating complex regulatory environments. In Europe, where sustainable development is a priority, various regulations and standards influence the adoption of facade-integrated PV systems. Understanding these regulations is essential for architects, engineers, and developers who seek to implement these systems in compliance with local and international laws.
European Union Directives and Regulations
At the heart of Europe’s regulatory landscape for renewable energy is the European Union’s Renewable Energy Directive (RED), which sets binding targets for the share of energy from renewable sources in the EU’s overall energy mix. The directive mandates that member states, including Germany and Austria, must meet specific renewable energy targets by 2030, with an emphasis on increasing the use of solar energy, including building-integrated photovoltaics (BIPV).
The Energy Performance of Buildings Directive (EPBD) is another critical piece of legislation that directly impacts facade-integrated PV systems. The EPBD requires that all new buildings in the EU meet nearly zero-energy building (NZEB) standards, meaning that they must have very high energy performance, with the remaining energy needs being met by renewable sources. Facade-integrated PV systems are a key technology that can help buildings achieve these standards.
In addition to these overarching EU directives, specific regulations at the national level govern the integration of PV systems into buildings. These regulations cover aspects such as building codes, electrical safety standards, and fire safety requirements, all of which must be considered when designing and installing facade-integrated PV systems.
Regulations in Germany
Germany, as a leader in renewable energy, has implemented stringent regulations and incentives to promote the use of facade-integrated PV systems. The country’s Renewable Energy Sources Act (EEG) provides a legal framework for the expansion of renewable energy, including incentives such as feed-in tariffs and subsidies for solar energy installations.
Building codes in Germany also play a significant role in the integration of PV systems. For example, the EnEV (Energieeinsparverordnung) sets energy efficiency requirements for buildings, which include provisions for the use of renewable energy technologies like PV. Additionally, the KfW (Kreditanstalt für Wiederaufbau) bank offers low-interest loans and grants for energy-efficient construction projects, further incentivizing the adoption of facade-integrated PV systems.
Fire safety regulations are particularly important in Germany, where building codes require that PV systems, including those integrated into facades, meet specific fire resistance standards. These standards are designed to prevent the spread of fire and ensure the safety of building occupants.
Regulations in Austria
In Austria, the regulatory environment for facade-integrated PV systems is similarly supportive, with a focus on meeting the country’s ambitious renewable energy goals. The Austrian Climate and Energy Strategy outlines the country’s commitment to achieving 100% renewable electricity by 2030, a goal that relies heavily on the expansion of solar energy, including BIPV.
Austrian building codes, such as the OIB-Richtlinien (Austrian Institute of Construction Engineering Guidelines), include specific requirements for energy efficiency and the use of renewable energy in buildings. These codes are aligned with the EU’s NZEB standards and encourage the integration of PV systems into new and existing buildings.
In terms of financial incentives, Austria offers various subsidies and grants for solar energy installations, including facade-integrated PV systems. These incentives are administered at both the federal and regional levels, with programs designed to support the adoption of renewable energy in urban areas.
Impact of Regulations on Design and Implementation
The regulatory frameworks in Germany, Austria, and the broader European Union have a direct impact on the design and implementation of facade-integrated PV systems. Compliance with these regulations is essential, not only for legal reasons but also to ensure the safety, efficiency, and economic viability of the systems.
For architects and engineers, understanding the regulatory environment is crucial during the design phase. This includes ensuring that the PV modules meet all relevant standards for energy efficiency, electrical safety, and fire resistance. Additionally, the need to comply with building codes may influence decisions related to the placement, orientation, and type of PV technology used.
Developers must also consider the impact of regulations on project timelines and costs. Navigating the permitting process, securing subsidies, and ensuring compliance with building codes can add complexity to the project but are necessary steps to ensure the successful integration of PV systems into building facades.
4.2 Economic Viability
The economic viability of facade-integrated PV systems is a key consideration for architects, developers, and building owners. While the initial investment in these systems can be significant, the long-term financial benefits, including energy savings, increased property value, and potential revenue from surplus energy, can make them a worthwhile investment. This section explores the economic factors that influence the adoption of facade-integrated PV systems, including cost analysis, return on investment (ROI), and available financial incentives.
Cost Analysis
The cost of facade-integrated PV systems can vary widely depending on several factors, including the type of PV technology used, the complexity of the installation, and the specific design requirements of the building. In general, facade-integrated PV systems tend to be more expensive than traditional rooftop installations due to the additional design and engineering work required to integrate the modules into the building envelope.
Key cost components of facade-integrated PV systems include:
- PV Modules: The cost of the PV modules themselves can vary based on the type of technology (monocrystalline, polycrystalline, thin-film) and the level of customization required for aesthetic integration. Custom-colored or semi-transparent modules typically command a premium price.
- Mounting Systems: Facade-integrated PV systems require specialized mounting systems that secure the modules to the building facade while maintaining structural integrity. The design of these systems can be complex, particularly for buildings with irregular or curved facades, adding to the overall cost.
- Inverters and Electrical Infrastructure: The cost of inverters, wiring, and other electrical components is another significant factor. For large installations, centralized inverters may be used, while smaller or more complex systems may require multiple micro-inverters or power optimizers, which can increase costs.
- Design and Installation: The design and installation of facade-integrated PV systems require specialized expertise, particularly in urban environments where space is limited and access may be challenging. The need for customized designs, careful planning to avoid shading, and compliance with building codes all contribute to higher installation costs compared to traditional PV systems.
Return on Investment (ROI)
Despite the higher upfront costs, facade-integrated PV systems can offer attractive returns on investment (ROI) over the long term. The primary financial benefits of these systems include energy savings, increased property value, and potential revenue from selling surplus electricity back to the grid.
- Energy Savings: The most direct financial benefit of facade-integrated PV systems is the reduction in energy costs. By generating electricity on-site, building owners can reduce their reliance on external energy sources, leading to significant savings over time. The extent of these savings depends on the efficiency of the PV system, the building’s energy needs, and local energy prices.
- Increased Property Value: Buildings with facade-integrated PV systems are often viewed as more desirable, particularly in markets where sustainability and energy efficiency are valued. The presence of a PV system can increase the property’s value by reducing operating costs and enhancing its appeal to environmentally conscious buyers or tenants.
- Revenue from Surplus Energy: In many European countries, including Germany and Austria, building owners can sell surplus electricity generated by their PV systems back to the grid, providing an additional revenue stream. Feed-in tariffs or other incentive programs may offer favorable rates for this electricity, further improving the ROI.
Financial Incentives and Subsidies
Financial incentives and subsidies play a crucial role in making facade-integrated PV systems economically viable. Both Germany and Austria offer a range of incentives designed to offset the initial costs of PV installations and encourage the adoption of renewable energy technologies.
- Germany: Under the Renewable Energy Sources Act (EEG), building owners can benefit from feed-in tariffs, which guarantee a fixed price for electricity generated by PV systems and fed back into the grid. Additionally, the KfW bank offers loans and grants for energy-efficient construction projects, including those that incorporate facade-integrated PV systems.
- Austria: Austria offers various subsidies at both the federal and regional levels to support solar energy installations. These subsidies can cover a significant portion of the installation costs, making facade-integrated PV systems more affordable. Programs such as the Klima- und Energiefonds (Climate and Energy Fund) provide grants for innovative energy projects, including facade-integrated PV systems.
Cost-Benefit Analysis
A cost-benefit analysis is an essential tool for evaluating the economic viability of facade-integrated PV systems. This analysis should consider both the upfront costs and the long-term financial benefits, including energy savings, increased property value, and potential revenue from surplus energy.
For example, in a typical urban setting in Germany, a facade-integrated PV system may have a payback period of 10 to 15 years, depending on factors such as the system’s efficiency, local energy prices, and the availability of subsidies. While this payback period may be longer than that of traditional rooftop systems, the additional benefits of aesthetic integration, compliance with energy regulations, and enhanced property value can make the investment worthwhile.
In Austria, similar payback periods can be expected, particularly when taking into account the generous subsidies and incentives available for PV installations. The strong regulatory support for renewable energy in both countries further enhances the long-term economic outlook for facade-integrated PV systems.
4.3 Market Trends and Future Outlook
The market for facade-integrated PV systems is evolving rapidly, driven by advances in technology, increasing demand for sustainable buildings, and supportive regulatory environments. This section explores current market trends in Europe, particularly in Germany and Austria, and provides an outlook on the future of facade-integrated PV systems.
Growing Demand for Sustainable Buildings
As awareness of climate change and environmental sustainability grows, there is increasing demand for buildings that meet high standards of energy efficiency and reduce carbon emissions. Facade-integrated PV systems are well-positioned to meet this demand, offering a way to generate renewable energy while also enhancing the building’s aesthetic appeal.
In Germany and Austria, this demand is reflected in the growing number of buildings that incorporate BIPV systems. From residential complexes to commercial skyscrapers, the trend toward sustainable architecture is driving the adoption of facade-integrated PV systems across a wide range of building types.
Technological Innovations
Technological innovations are playing a crucial role in expanding the market for facade-integrated PV systems. Advances in PV materials, such as the development of perovskite-based cells, are leading to higher efficiencies and lower costs. These innovations are making facade-integrated PV systems more competitive with traditional rooftop systems, particularly in urban environments where space is limited.
The development of semi-transparent and flexible PV modules is also opening up new possibilities for integration into building facades. These technologies allow for the seamless incorporation of PV modules into windows, curtain walls, and other architectural elements, making them an attractive option for modern buildings.
Regulatory Support and Market Growth
Regulatory support for renewable energy continues to be a major driver of market growth for facade-integrated PV systems. The European Union’s commitment to achieving its renewable energy targets, coupled with national incentives in Germany and Austria, is creating a favorable environment for the adoption of these systems.
As building codes and energy standards become more stringent, the role of facade-integrated PV systems in achieving compliance is likely to increase. This trend is expected to drive further growth in the market, particularly in countries like Germany and Austria, where sustainability is a key priority.
Challenges and Opportunities
While the market for facade-integrated PV systems is growing, there are still challenges to be addressed. The higher upfront costs, complexity of installation, and need for specialized expertise can be barriers to adoption. Additionally, the integration of PV systems into existing buildings can be more challenging than in new constructions, requiring careful planning and coordination.
However, these challenges also present opportunities for innovation. The development of new materials, improved installation techniques, and more efficient PV technologies can help overcome these barriers and drive further market growth. Additionally, the increasing demand for sustainable buildings is likely to continue fueling interest in facade-integrated PV systems, creating new opportunities for architects, engineers, and developers.
Future Outlook
The future of facade-integrated PV systems looks promising, particularly in Europe, where regulatory support and market demand are strong. As technology continues to advance and costs decrease, these systems are likely to become an increasingly common feature of urban architecture.
In Germany and Austria, the commitment to renewable energy and sustainable building practices suggests that the adoption of facade-integrated PV systems will continue to grow. With the right combination of regulatory support, technological innovation, and market demand, facade-integrated PV systems have the potential to play a significant role in the transition to a sustainable energy future.
Regulatory and economic considerations are critical to the successful adoption of facade-integrated PV systems. Understanding the regulatory landscape, evaluating the economic viability, and staying informed about market trends are essential for architects, engineers, and developers looking to implement these systems. The strong regulatory support in Europe, particularly in Germany and Austria, combined with technological innovations and growing market demand, suggests a bright future for facade-integrated PV systems. The next and final section will focus on case studies and best practices, providing concrete examples of how these systems have been successfully implemented in real-world projects.
Section 5: Case Studies and Best Practices
5.1 Successful Implementation Examples in Germany and Austria
The integration of photovoltaic (PV) modules into building facades has seen notable success in Germany and Austria, where sustainable architecture and renewable energy are national priorities. This section explores several case studies of buildings in these countries that have successfully incorporated facade-integrated PV systems, demonstrating both the potential and the challenges of this technology.
Case Study 1: The BIQ House, Hamburg, Germany
The BIQ House in Hamburg is one of the most innovative examples of facade-integrated PV systems in Europe. This building, also known as the “algae house,” is part of the International Building Exhibition (IBA) and features a bio-adaptive facade that includes both PV modules and algae bioreactors.
The PV modules in the BIQ House are integrated into the south-facing facade, capturing sunlight to generate electricity for the building. The design of the facade is highly innovative, combining energy generation with other sustainable technologies. The building uses the electricity generated by the PV modules to power its systems, reducing its reliance on external energy sources.
One of the key challenges in the design of the BIQ House was balancing the aesthetic appeal of the facade with the functional requirements of the PV system. The architects successfully addressed this by using custom-colored PV modules that blend seamlessly with the building’s overall design. The result is a building that not only generates energy but also stands as a striking example of modern sustainable architecture.
Case Study 2: The Raiffeisen Tower, Vienna, Austria
The Raiffeisen Tower in Vienna is another exemplary project that showcases the successful integration of PV modules into a building facade. This high-rise office building is one of the tallest in Vienna and is known for its distinctive curvilinear design, which incorporates facade-integrated PV systems into its surface.
The PV modules are integrated into the building’s glass curtain wall, creating a semi-transparent facade that allows natural light to enter the building while generating electricity. The use of semi-transparent modules was a key design choice, enabling the building to maintain its sleek, modern appearance while contributing to its energy efficiency.
The Raiffeisen Tower was designed to meet the highest standards of energy efficiency and sustainability. The PV system is an integral part of this approach, providing a significant portion of the building’s electricity needs and reducing its carbon footprint. The project demonstrates how facade-integrated PV systems can be used in large-scale commercial buildings, offering both environmental and economic benefits.
Case Study 3: The Solar City, Linz, Austria
Solar City in Linz is a pioneering project in sustainable urban development, featuring extensive use of facade-integrated PV systems across multiple buildings. This planned community was designed with the goal of creating a self-sufficient, zero-energy district, and PV technology plays a central role in achieving this vision.
The buildings in Solar City incorporate PV modules into their facades, roofs, and even shading devices. The integration of PV systems into the building facades allows for the generation of electricity in areas where traditional rooftop installations would be impractical or insufficient. The design of the buildings emphasizes the harmonious integration of renewable energy systems with the urban environment, creating a community that is both aesthetically pleasing and highly energy-efficient.
One of the key lessons from Solar City is the importance of planning and coordination in the design and implementation of facade-integrated PV systems. The success of the project was made possible by close collaboration between architects, engineers, and urban planners, ensuring that the PV systems were effectively integrated into the overall design of the buildings and the community.
5.2 Innovative Approaches and Emerging Technologies
As the demand for sustainable buildings grows, architects and engineers are exploring innovative approaches and emerging technologies to enhance the performance and aesthetics of facade-integrated PV systems. This section highlights some of the most promising developments in the field, focusing on technologies that are likely to shape the future of facade-integrated PV.
Bifacial PV Modules
Bifacial PV modules, which can capture sunlight from both the front and back sides, are an emerging technology with significant potential for facade integration. These modules are particularly effective in urban environments where sunlight is often reflected off surrounding buildings and surfaces. By capturing both direct and reflected light, bifacial modules can increase energy generation, making them an attractive option for facade applications.
In Germany and Austria, several projects have begun to explore the use of bifacial modules in building facades. These projects demonstrate the potential of this technology to enhance the efficiency of facade-integrated PV systems, particularly in densely built environments where maximizing energy generation is critical.
Semi-Transparent and Transparent PV Modules
The development of semi-transparent and fully transparent PV modules is another key innovation that is transforming the design of facade-integrated PV systems. These modules allow for the integration of PV technology into windows, skylights, and glass curtain walls, enabling buildings to generate electricity while still allowing natural light to enter.
Semi-transparent PV modules are particularly well-suited for buildings with large glass facades, such as office towers and commercial buildings. In addition to generating electricity, these modules can also provide shading, reducing the building’s cooling load and improving its overall energy efficiency.
One notable example of this technology in use is the Copenhagen International School in Denmark, which features a facade composed of more than 12,000 blue-tinted, semi-transparent PV modules. While not in Germany or Austria, this project has garnered international attention and serves as an inspiration for similar developments in those countries.
Building-Integrated Photovoltaics (BIPV) Glass
Building-integrated photovoltaic glass is a rapidly developing technology that combines the functions of traditional glass with the ability to generate electricity. BIPV glass can be used in windows, curtain walls, and other glass surfaces, making it a versatile option for integrating PV systems into building facades.
In Austria, the use of BIPV glass has been explored in several pilot projects, with promising results. The technology offers a way to seamlessly incorporate PV systems into the building envelope without compromising aesthetics or functionality. As the technology continues to evolve, it is likely to become an increasingly popular choice for facade-integrated PV systems.
Flexible and Lightweight PV Modules
Flexible and lightweight PV modules are another innovation that holds promise for facade integration. These modules can be applied to curved or irregular surfaces, offering new possibilities for integrating PV systems into complex architectural designs. Their lightweight nature also makes them suitable for retrofitting existing buildings, where structural limitations may prevent the use of traditional PV modules.
Several projects in Germany have begun to explore the use of flexible PV modules in facade applications. These projects highlight the potential of this technology to expand the range of buildings that can benefit from facade-integrated PV systems, including older buildings that may not be able to support the weight of traditional modules.
5.3 Comparative Analysis with Other Regions
While Europe, particularly Germany and Austria, has been a leader in the adoption of facade-integrated PV systems, other regions around the world are also exploring this technology. This section provides a comparative analysis of facade-integrated PV systems in Europe versus other regions, highlighting the unique approaches and lessons that can be drawn from these global examples.
North America
In North America, the adoption of facade-integrated PV systems has been slower than in Europe, largely due to differences in regulatory environments and market demand. However, there are several notable projects in the United States and Canada that demonstrate the potential of this technology.
One such example is the Solar Carve Tower in New York City, which features a diamond-shaped facade that integrates PV modules. This project highlights the potential for innovative architectural designs that incorporate PV technology, even in densely built urban environments. However, the lack of strong regulatory support and financial incentives has limited the widespread adoption of facade-integrated PV systems in North America compared to Europe.
Asia
In Asia, the adoption of facade-integrated PV systems is growing rapidly, particularly in countries like China, Japan, and South Korea. These countries have embraced PV technology as part of their broader efforts to reduce carbon emissions and increase energy efficiency.
In China, for example, the Tianjin Binhai Library features a facade-integrated PV system that generates electricity while also providing shading for the building’s interior. This project demonstrates the potential for large-scale adoption of facade-integrated PV systems in rapidly growing urban environments. The strong government support for renewable energy in China has been a key factor in driving the adoption of this technology.
Middle East
The Middle East presents a unique environment for facade-integrated PV systems due to its high levels of solar radiation and growing demand for sustainable buildings. In the United Arab Emirates, for example, the Dubai Sustainable City development features buildings with facade-integrated PV systems that help to meet the energy needs of the community.
While the Middle East has favorable conditions for PV technology, challenges such as high temperatures and dust accumulation can impact the efficiency of facade-integrated systems. However, ongoing research and innovation are addressing these challenges, making the region an important area for the development of facade-integrated PV technology.
Lessons and Best Practices
The comparative analysis of facade-integrated PV systems in different regions highlights several key lessons and best practices that can inform future projects in Germany, Austria, and beyond:
- Regulatory Support: Strong regulatory support is crucial for the widespread adoption of facade-integrated PV systems. Countries that offer clear guidelines, incentives, and subsidies have seen higher rates of adoption and more successful projects.
- Technological Innovation: The development and application of new PV technologies, such as bifacial modules and BIPV glass, are essential for expanding the possibilities of facade integration. These innovations allow for greater flexibility in design and improved efficiency, making PV systems more attractive for a wider range of buildings.
- Collaboration: Successful facade-integrated PV projects often involve close collaboration between architects, engineers, and urban planners. This collaboration ensures that PV systems are effectively integrated into the building’s design and that they meet both aesthetic and functional requirements.
- Adaptation to Local Conditions: The performance of facade-integrated PV systems is heavily influenced by local environmental conditions, such as solar radiation, temperature, and shading. Adapting the design and technology of PV systems to these conditions is crucial for maximizing their efficiency and viability.
The case studies and comparative analysis presented in this section illustrate the potential of facade-integrated PV systems to transform urban architecture and contribute to sustainable development. By learning from successful projects in Germany, Austria, and other regions, architects, engineers, and developers can apply best practices and innovative approaches to future projects, ensuring that facade-integrated PV systems continue to play a vital role in the transition to renewable energy. As the technology evolves and market demand grows, facade-integrated PV systems are likely to become an increasingly common feature of buildings around the world, helping to create more sustainable and energy-efficient cities.
Section 6: Future Prospects and Conclusions
6.1 The Future of Facade-Integrated Photovoltaic Modules
Facade-integrated photovoltaic (PV) systems are poised to become a cornerstone of sustainable urban development. As cities continue to grow and the demand for renewable energy increases, these systems offer a viable solution for reducing carbon footprints while enhancing the aesthetic and functional aspects of buildings. The future of facade-integrated PV systems will be shaped by ongoing advancements in technology, evolving regulatory frameworks, and the growing recognition of the importance of sustainable design.
Technological Innovations Driving the Future
The future of facade-integrated PV systems will be heavily influenced by technological innovations that improve efficiency, reduce costs, and expand the range of applications. Key areas of innovation include:
- Advanced PV Materials: Research into new materials, such as perovskites and organic photovoltaics, promises to increase the efficiency and reduce the cost of PV modules. These materials could enable the production of lighter, more flexible, and more efficient PV modules, making it easier to integrate them into building facades.
- BIPV Glass and Transparent Modules: The development of building-integrated photovoltaic (BIPV) glass and transparent modules will continue to expand the possibilities for integrating PV systems into windows, skylights, and other glass surfaces. These technologies allow for the seamless incorporation of PV systems into the design of modern buildings without compromising natural light or aesthetic appeal.
- Energy Storage Solutions: As facade-integrated PV systems generate electricity throughout the day, the integration of energy storage solutions will become increasingly important. Advances in battery technology, including the development of more efficient and longer-lasting batteries, will enable buildings to store excess energy generated by PV systems for use during periods of low sunlight or peak demand.
- Smart Building Integration: The future of facade-integrated PV systems will also involve their integration into smart building technologies. By connecting PV systems to building management systems, it will be possible to optimize energy use, monitor performance in real-time, and respond to changing conditions, such as shading or weather, to maximize efficiency.
Evolving Regulatory Frameworks
As the adoption of facade-integrated PV systems grows, regulatory frameworks will continue to evolve to support this technology. In Europe, the push toward nearly zero-energy buildings (NZEB) and the commitment to reducing greenhouse gas emissions will drive the development of new regulations that encourage the use of renewable energy in building design.
- Stricter Energy Efficiency Standards: Future regulations are likely to include stricter energy efficiency standards for new and existing buildings, making the integration of PV systems into facades not only desirable but necessary. These standards will encourage architects and developers to incorporate renewable energy systems into their designs from the outset.
- Incentives for Retrofits: As part of the transition to a more sustainable built environment, governments may introduce new incentives and subsidies for retrofitting existing buildings with facade-integrated PV systems. These incentives could help offset the cost of installation and encourage building owners to adopt this technology.
- Urban Planning and Zoning Regulations: Urban planning and zoning regulations may also evolve to support the use of facade-integrated PV systems. For example, cities may introduce requirements for solar-ready building designs or offer bonuses for developments that incorporate renewable energy systems into their facades.
Market Growth and Adoption
The market for facade-integrated PV systems is expected to grow significantly in the coming years, driven by the increasing demand for sustainable buildings and the need to meet renewable energy targets. This growth will be particularly strong in urban areas, where the pressure to reduce carbon emissions and improve energy efficiency is greatest.
- Expansion in Urban Areas: As cities become denser and the availability of rooftop space becomes more limited, the adoption of facade-integrated PV systems will expand. These systems offer a way to generate renewable energy in environments where traditional solar installations may not be feasible.
- Rising Consumer Awareness: As awareness of climate change and the importance of sustainability continues to grow, consumers will increasingly demand buildings that incorporate renewable energy systems. This demand will drive the adoption of facade-integrated PV systems in both residential and commercial developments.
- Collaboration Across Sectors: The successful implementation of facade-integrated PV systems will require collaboration across sectors, including architecture, engineering, urban planning, and government. This collaboration will be essential for overcoming the challenges associated with integrating PV systems into building facades and ensuring that they deliver the desired environmental and economic benefits.
6.2 Conclusion: The Role of Facade-Integrated PV Systems in Sustainable Development
Facade-integrated PV systems represent a critical innovation in the quest for sustainable urban development. By harnessing the power of the sun to generate electricity, these systems offer a way to reduce carbon emissions, improve energy efficiency, and contribute to the aesthetic and functional qualities of buildings.
The case studies and examples from Germany, Austria, and other regions demonstrate the potential of facade-integrated PV systems to transform the way we design and power our buildings. These systems have already proven their value in a range of applications, from residential buildings to commercial skyscrapers, and their adoption is expected to grow as technology advances and regulatory support increases.
For architects, engineers, and developers, the integration of PV modules into building facades offers a unique opportunity to contribute to the global effort to combat climate change. By embracing this technology, they can help create buildings that are not only beautiful and functional but also aligned with the principles of sustainability.
Key Takeaways:
- Sustainable Energy Generation: Facade-integrated PV systems enable buildings to generate renewable energy on-site, reducing reliance on external energy sources and contributing to the global transition to sustainable energy.
- Architectural Flexibility: These systems offer a high degree of design flexibility, allowing architects to integrate PV modules into a wide range of building styles and materials without compromising aesthetics.
- Economic and Regulatory Benefits: The economic viability of facade-integrated PV systems is supported by a range of incentives and subsidies, particularly in Europe. As regulatory frameworks continue to evolve, the adoption of these systems is expected to become more widespread.
- Future Growth and Innovation: The future of facade-integrated PV systems will be shaped by ongoing technological innovation, evolving regulations, and growing market demand. As these systems become more efficient, cost-effective, and easier to integrate, they will play an increasingly important role in sustainable urban development.
In conclusion, facade-integrated PV systems offer a powerful solution for addressing the energy challenges of the 21st century. By integrating renewable energy generation into the very fabric of our buildings, we can create a more sustainable future for our cities and our planet. The journey toward widespread adoption of this technology is well underway, and the prospects for its continued growth and development are bright. As we look to the future, facade-integrated PV systems will undoubtedly be a key component of the sustainable cities of tomorrow.