This comprehensive guide explores the key components of photovoltaic systems, focusing on their optimal configuration for various installation types, with a particular emphasis on applications in Germany and Austria. The guide begins with an overview of PV technology, examining the evolution of solar modules, including monocrystalline, polycrystalline, and thin-film technologies, and their relevance to European climates. Detailed attention is given to the role of inverters, covering string, central, and microinverters, and their critical specifications such as efficiency and grid compatibility.
The discussion extends to energy storage solutions, highlighting the importance of battery technology, including lithium-ion and emerging systems, in enhancing system reliability and energy independence. Additionally, the guide addresses the balance of system (BOS) components, essential for optimizing overall system efficiency, and offers best practices for system design and configuration, integrating considerations for grid-tied and off-grid applications. Case studies from Germany and Austria illustrate real-world applications, while future trends, including AI and IoT integration, are explored to forecast the evolving landscape of PV systems in Europe. This guide serves as both a technical resource and a strategic tool for engineers and managers involved in the renewable energy sector. By Alireza Alidadi, Cademix Institute of Technology
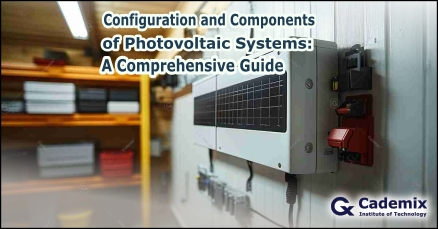
Introduction
Photovoltaic (PV) systems have become an integral part of the global energy landscape, with their adoption rapidly increasing, particularly in Europe. These systems harness the power of the sun to generate electricity, offering a sustainable alternative to traditional fossil fuels. As the demand for renewable energy solutions grows, so does the need for a comprehensive understanding of the components that make up PV systems and how they can be configured for optimal performance.
This guide is designed to provide a deep dive into the critical components of PV systems, including solar modules, inverters, and energy storage solutions. It aims to equip engineers, technical managers, and decision-makers with the knowledge necessary to make informed decisions about system design and configuration. The focus is on practical applications, particularly within the context of Germany and Austria, where the regulatory environment and market dynamics offer unique challenges and opportunities.
The author, with extensive experience in the field, has been involved in several successful PV projects, as illustrated in Figure 1. These projects have provided valuable insights into the practical aspects of PV system implementation, from selecting the right components to optimizing system configurations for different installation types. This experience underpins the recommendations and strategies presented in this guide, ensuring they are grounded in real-world applications.
Understanding the components of a PV system is essential not only for engineers but also for general managers and decision-makers who may not have a technical background. This guide is therefore written in an accessible manner, avoiding unnecessary jargon while still providing the depth of detail needed for those involved in the design and implementation of these systems.
The structure of this guide is straightforward, beginning with an overview of PV systems and their core components. From there, it delves into the specifics of solar modules, inverters, and energy storage solutions, followed by a discussion on balance of system (BOS) components and system configuration strategies. Throughout, examples from Germany and Austria are used to illustrate key points and provide context.
As the renewable energy sector continues to evolve, staying informed about the latest technologies and best practices is crucial. This guide is intended to be both a resource and a strategic tool, helping professionals in the field to navigate the complexities of PV systems and to contribute effectively to the transition toward sustainable energy.
1.Overview of Photovoltaic Systems and Solar Modules
Photovoltaic (PV) systems are at the forefront of the global shift towards renewable energy. By converting sunlight into electricity, PV systems provide a clean, sustainable alternative to conventional power sources. This section explores the foundational aspects of PV systems, with a focus on solar modules—the most critical component in the conversion process.
1.1 Introduction to Components of Photovoltaic Systems
At their core, PV systems operate by capturing sunlight and converting it into electrical energy through the photovoltaic effect. This process occurs when photons from sunlight strike the surface of a semiconductor material, typically silicon, within a solar cell. The energy from the photons dislodges electrons in the semiconductor, creating an electric current. This current is then collected and used to power electrical devices or fed into the grid.
PV systems can be deployed in various configurations, ranging from small residential installations to large utility-scale solar farms. The specific configuration depends on factors such as available space, energy requirements, and local regulations. In Europe, particularly in countries like Germany and Austria, PV systems are often integrated into both residential and commercial buildings, taking advantage of incentives and favorable regulatory frameworks.
1.2 Solar Modules: The Heart of Photovoltaic Systems
Solar modules, or panels, are the most visible component of a PV system. They consist of multiple solar cells connected together to increase the overall electrical output. The performance and efficiency of a PV system largely depend on the type and quality of the solar modules used.
There are three primary types of solar modules: monocrystalline, polycrystalline, and thin-film. Each type has its own set of characteristics, advantages, and limitations.
- Monocrystalline Modules: These are made from a single crystal structure, typically silicon. Monocrystalline modules are known for their high efficiency, often exceeding 20%, and their long lifespan. They perform well in a range of conditions, including low-light environments, which makes them particularly suitable for regions with variable weather patterns, such as Germany and Austria. However, they tend to be more expensive than other types of modules.
- Polycrystalline Modules: Polycrystalline modules are made from silicon crystals that have been melted together. While slightly less efficient than monocrystalline modules, typically ranging from 15% to 18%, they are more cost-effective and have a shorter energy payback time. This makes them a popular choice for large-scale installations where cost is a significant consideration.
- Thin-Film Modules: These modules are made by depositing one or more thin layers of photovoltaic material onto a substrate. Thin-film technology includes various materials, such as cadmium telluride (CdTe) and copper indium gallium selenide (CIGS). Thin-film modules are generally less efficient than crystalline silicon modules, with efficiencies ranging from 10% to 12%. However, they are lightweight, flexible, and can be produced in large sizes, making them ideal for certain applications, such as building-integrated photovoltaics (BIPV).
1.3 Key Parameters Affecting Solar Module Performance
When selecting solar modules, it’s crucial to consider key performance parameters that impact the overall efficiency and long-term viability of the PV system. These parameters include:
- Efficiency: This measures how well a solar module converts sunlight into usable electricity. Higher efficiency modules produce more power per square meter, which is particularly important in areas with limited installation space.
- Temperature Coefficient: This parameter indicates how the efficiency of a solar module decreases as the temperature rises. Modules with a lower temperature coefficient are better suited for regions with high ambient temperatures, as they maintain higher efficiency under heat.
- Degradation Rate: Over time, solar modules lose efficiency due to environmental factors such as UV exposure, temperature fluctuations, and mechanical stress. The degradation rate is the rate at which a module’s performance declines each year. Modules with lower degradation rates offer more reliable long-term performance.
1.4 Innovations in Solar Module Technology
The field of photovoltaic technology is constantly evolving, with new innovations aimed at improving efficiency, reducing costs, and expanding the applications of solar energy. Some of the notable advancements include:
- Bifacial Modules: These modules capture sunlight on both sides, increasing the overall energy yield, especially in environments with high albedo surfaces like snow or white rooftops.
- PERC Technology: Passivated Emitter and Rear Cell (PERC) technology adds an additional layer to the back of the solar cell, allowing it to capture more light and improve efficiency.
- Building-Integrated Photovoltaics (BIPV): BIPV involves integrating solar modules into the building materials, such as roof tiles or façades, providing both energy generation and aesthetic appeal.
1.5 Real-World Applications: Germany and Austria
In Germany and Austria, solar modules are a common sight on residential rooftops, commercial buildings, and even agricultural lands. These countries have embraced solar energy as part of their broader renewable energy strategies, driven by favorable policies and public support. For example, Germany’s Renewable Energy Sources Act (EEG) has provided significant incentives for solar installations, leading to widespread adoption.
In Austria, similar incentives and a strong focus on sustainability have resulted in a growing number of solar installations. Both countries serve as leading examples of how solar modules can be effectively integrated into various settings to provide clean, renewable energy.
2.Inverters: The Powerhouse of Photovoltaic Systems
Inverters are a critical component of photovoltaic (PV) systems, acting as the intermediary between the solar modules and the electrical grid or the load. Their primary function is to convert the direct current (DC) produced by solar modules into alternating current (AC), which is the form of electricity used by most appliances and the power grid. This section delves into the types of inverters, their key specifications, and innovations that are shaping the future of PV systems.
2.1 The Role of Inverters in Photovoltaic Systems
Inverters play a vital role in ensuring that the electricity generated by solar modules can be effectively used or stored. Without an inverter, the DC electricity produced by the panels would be incompatible with most household appliances and the grid. In addition to converting DC to AC, inverters are responsible for several other crucial functions:
- Maximum Power Point Tracking (MPPT): MPPT is a technique used by inverters to maximize the power output from the solar modules. Solar modules operate most efficiently at a specific voltage and current, known as the maximum power point. The inverter continuously adjusts the electrical load to keep the modules operating at this optimal point, thus maximizing energy harvest.
- Grid Synchronization: For grid-tied PV systems, inverters must synchronize the output AC with the grid’s frequency and voltage. This ensures that the power generated by the PV system can be safely and efficiently fed into the grid.
- Monitoring and Diagnostics: Modern inverters come with built-in monitoring systems that track the performance of the PV system. This includes real-time data on power output, efficiency, and potential issues. Some inverters also offer remote monitoring capabilities, allowing users to keep track of their system’s performance from anywhere.
2.2 Types of Inverters
Inverters come in various types, each suited to different system configurations and installation scenarios. The choice of inverter type can significantly impact the efficiency, reliability, and cost of a PV system.
- String Inverters: String inverters are the most common type of inverter used in residential and small commercial PV systems. In this setup, multiple solar modules are connected in series to form a “string,” and the DC output from the string is fed into the inverter. String inverters are cost-effective and relatively simple to install. However, their performance can be affected by shading, as the entire string’s output is limited by the lowest-performing module.
- Central Inverters: Central inverters are larger and more powerful than string inverters, making them suitable for large commercial and utility-scale PV installations. They operate similarly to string inverters but on a much larger scale, handling the output of multiple strings of solar modules. Central inverters offer high efficiency and are often more economical for large projects, but they require more space and can be challenging to maintain.
- Microinverters: Unlike string and central inverters, microinverters are small devices installed on each solar module. They convert DC to AC at the module level, which allows each module to operate independently. This setup minimizes the impact of shading or module mismatch on the overall system performance. Microinverters are ideal for installations with complex layouts or partial shading. While they offer improved energy harvest and system reliability, they are generally more expensive and require more installation time.
2.3 Key Specifications of Inverters
When selecting an inverter, several key specifications must be considered to ensure that the PV system operates efficiently and reliably over its lifetime.
- Efficiency: Inverter efficiency refers to the percentage of DC electricity that is successfully converted to AC. Modern inverters typically have efficiencies ranging from 95% to 99%. Higher efficiency inverters reduce energy losses and increase the overall energy yield of the system.
- Maximum Power Point Tracking (MPPT) Range: The MPPT range defines the voltage window within which the inverter can effectively track the maximum power point of the solar modules. A wider MPPT range allows the inverter to operate efficiently across a broader range of conditions, such as varying sunlight and temperature.
- Power Rating: The power rating of an inverter indicates the maximum amount of AC power it can deliver. It is essential to match the inverter’s power rating with the size of the PV system to avoid underutilization or overloading.
- Grid Compatibility: Inverters must be compatible with the local grid standards, including voltage and frequency requirements. In regions like Germany and Austria, where grid stability and compliance are critical, selecting an inverter that meets local standards is essential.
2.4 Innovations in Inverter Technology
Inverter technology is continuously evolving, with new advancements aimed at improving efficiency, reliability, and integration with other energy systems. Some of the most significant innovations include:
- Smart Inverters: Smart inverters are equipped with advanced features that allow them to interact with the grid more intelligently. They can provide grid support functions, such as voltage regulation and reactive power control, helping to maintain grid stability as the penetration of PV systems increases. Smart inverters are becoming increasingly important in markets with high levels of solar adoption, such as Germany.
- Hybrid Inverters: Hybrid inverters can manage multiple power sources, such as solar panels and batteries, within a single system. They are ideal for installations that include energy storage, as they can control the flow of electricity between the solar modules, the battery, and the grid. Hybrid inverters are essential for optimizing self-consumption and enabling grid independence in both residential and commercial settings.
- Integrated Monitoring and IoT Connectivity: Modern inverters often come with integrated monitoring systems and Internet of Things (IoT) connectivity. These features allow for real-time performance tracking, remote diagnostics, and even predictive maintenance. By analyzing data from multiple inverters, operators can optimize the performance of large-scale installations and reduce downtime.
2.5 Real-World Applications: Germany and Austria
Germany and Austria have been at the forefront of adopting advanced inverter technologies, driven by robust renewable energy policies and a strong focus on grid stability. In these countries, smart inverters are commonly used in both residential and commercial PV systems to comply with grid requirements and maximize energy yield.
For example, in Germany, the Renewable Energy Sources Act (EEG) mandates the use of inverters that can support grid stability functions, which has accelerated the adoption of smart inverter technology. In Austria, the focus on self-consumption has led to widespread use of hybrid inverters in residential settings, enabling homeowners to maximize the use of solar energy and reduce reliance on the grid.
In both countries, the integration of monitoring and IoT connectivity has become standard practice, allowing system operators to optimize performance and ensure the long-term viability of their PV installations.
3.Energy Storage Solutions: Enhancing the Reliability of Photovoltaic Systems
As photovoltaic (PV) systems continue to proliferate, the need for reliable energy storage solutions has become increasingly critical. Energy storage not only helps balance the intermittent nature of solar power but also enhances the overall efficiency and flexibility of PV systems. This section explores the importance of energy storage, the different types of storage systems available, key configuration considerations, and real-world applications in Germany and Austria.
3.1 The Importance of Energy Storage in Photovoltaic Systems
Energy storage plays a vital role in overcoming one of the main challenges associated with solar energy: its variability. Solar power generation is inherently dependent on sunlight, which means that energy production fluctuates throughout the day and is nonexistent at night. Without storage, excess energy generated during peak sunlight hours cannot be used later when demand may be higher.
By integrating energy storage solutions, PV systems can store excess energy produced during the day and release it when needed, such as during the evening or during periods of low sunlight. This capability not only improves the reliability of the energy supply but also enhances self-consumption, reducing reliance on the grid. In regions like Germany and Austria, where self-consumption is increasingly encouraged, energy storage systems are becoming a key component of both residential and commercial PV installations.
3.2 Types of Energy Storage Systems
There are several types of energy storage systems used in conjunction with PV systems, each with its own set of advantages and limitations. The choice of storage system depends on factors such as the size of the PV installation, energy demand patterns, and budget.
- Lead-Acid Batteries: Lead-acid batteries are one of the oldest and most widely used types of energy storage. They are relatively inexpensive and have a proven track record of reliability. However, lead-acid batteries have a lower energy density compared to newer technologies, which means they require more space for the same amount of stored energy. They also have a shorter lifespan and lower efficiency, typically around 80% to 85%. Despite these limitations, lead-acid batteries remain a viable option for off-grid systems or situations where cost is a primary concern.
- Lithium-Ion Batteries: Lithium-ion batteries have emerged as the preferred choice for modern energy storage solutions, particularly in residential and commercial PV systems. They offer higher energy density, longer lifespan, and better efficiency, often exceeding 95%. Lithium-ion batteries are also more compact and lighter, making them suitable for a wide range of applications. However, they are more expensive than lead-acid batteries, which can be a consideration for larger systems. Their superior performance and decreasing costs have made them the dominant technology in new installations, especially in markets like Germany and Austria.
- Flow Batteries: Flow batteries are an emerging technology that offers unique advantages for large-scale energy storage. Unlike conventional batteries, flow batteries store energy in liquid electrolytes, which are housed in external tanks. This design allows for easy scalability—adding more storage capacity is simply a matter of increasing the size of the tanks. Flow batteries have a long cycle life and can be discharged completely without damaging the system, making them ideal for large installations with high energy demands. However, they are still relatively expensive and complex, which limits their adoption to specific applications.
- Other Emerging Technologies: In addition to these mainstream options, several other energy storage technologies are under development, including solid-state batteries, hydrogen storage, and compressed air energy storage (CAES). While these technologies hold promise for the future, they are still in the early stages of commercial deployment and are not yet widely used in conjunction with PV systems.
3.3 Configuration Considerations for Energy Storage
Proper configuration of energy storage systems is essential to maximize the benefits of a PV installation. Several factors must be considered when designing a storage solution, including the size of the storage system, the relationship between the PV array and storage capacity, and the intended use of the stored energy.
- Sizing of Storage Systems: The size of the storage system should be carefully matched to the energy production and consumption patterns of the site. If the storage system is too small, it will not be able to capture all of the excess energy generated by the PV system, leading to wasted potential. Conversely, if the storage system is too large, it may represent an unnecessary expense and could remain underutilized. The ideal size depends on factors such as daily energy consumption, peak demand periods, and the availability of sunlight.
- PV Array and Storage Capacity: The relationship between the PV array size and storage capacity is crucial. In general, a larger PV array can generate more energy, but this energy needs to be stored efficiently to be useful during non-production hours. Configurations where the storage capacity is aligned with the output of the PV array ensure that the system operates at optimal efficiency, minimizing energy losses and maximizing return on investment.
- Use Cases for Stored Energy: The intended use of stored energy also influences the configuration of the storage system. For example, a system designed to maximize self-consumption will prioritize discharging stored energy during periods of high demand or when grid electricity is most expensive. In contrast, a system intended for grid independence might focus on ensuring a stable power supply during outages, requiring a different storage strategy.
- Grid Interaction and Regulations: In grid-tied systems, the interaction between the energy storage system and the grid must be considered. In many regions, including Germany and Austria, regulations govern how and when stored energy can be fed back into the grid. These regulations can impact the design and operation of the storage system, particularly in terms of grid stability and compliance with local standards.
3.4 Real-World Applications: Germany and Austria
Germany and Austria are leading examples of how energy storage solutions are being integrated into PV systems to enhance their efficiency and reliability. Both countries have adopted policies that encourage the use of energy storage, particularly in conjunction with residential PV installations.
In Germany, the combination of generous feed-in tariffs and the decreasing cost of lithium-ion batteries has led to a significant increase in the adoption of residential energy storage systems. Homeowners are increasingly installing batteries to store excess solar energy, which they can then use during the evening or sell back to the grid. This trend has been supported by government incentives, such as the KfW Development Bank’s subsidy program, which offers financial support for battery storage systems.
Austria has also seen a rise in the use of energy storage, driven by a strong emphasis on renewable energy and self-consumption. In Austria, the focus is on maximizing the use of solar energy within homes and businesses, reducing dependence on the grid, and lowering energy costs. Energy storage solutions, particularly lithium-ion batteries, have become a key part of this strategy, enabling users to take full advantage of their PV systems.
In both countries, energy storage systems are not only enhancing the efficiency and reliability of PV installations but also contributing to broader energy goals, such as reducing carbon emissions and increasing energy independence.
4.Balance of System (BOS) Components: Supporting the Core of Photovoltaic Systems
While solar modules, inverters, and energy storage solutions are the most visible and often discussed components of photovoltaic (PV) systems, they represent only part of the overall infrastructure. The Balance of System (BOS) components play a crucial role in ensuring that the entire system functions efficiently and safely. BOS components include everything that connects and supports the core elements of a PV system, such as mounting structures, wiring, junction boxes, and protection devices. This section provides a detailed overview of these components, their importance, and best practices for configuration, with a focus on applications in Germany and Austria.
4.1 Overview of BOS Components
BOS components are essential to the operation of any PV system. They provide the necessary infrastructure to connect solar modules to inverters and energy storage solutions, and ultimately to the grid or the load. While often overlooked, the quality and configuration of BOS components can significantly impact the overall performance, efficiency, and longevity of a PV system.
- Mounting Structures: Mounting structures are used to securely attach solar modules to various surfaces, such as rooftops, the ground, or building façades. These structures must be robust enough to withstand environmental factors like wind, snow, and temperature fluctuations, while also optimizing the angle and orientation of the modules to maximize sunlight exposure. In regions like Germany and Austria, where weather conditions can be challenging, selecting the right mounting structure is critical for ensuring the long-term stability and performance of the system.
- Wiring and Cabling: Wiring is the lifeline of a PV system, responsible for transmitting the electrical power generated by the solar modules to the inverter and beyond. The quality of the wiring, including the type, size, and insulation, directly affects the efficiency and safety of the system. Proper wiring minimizes energy losses due to resistance and ensures that the system can handle the electrical load without overheating or degrading over time. In Europe, where strict electrical standards are enforced, using high-quality, certified wiring is essential for compliance and reliability.
- Junction Boxes: Junction boxes are used to combine and route electrical connections within the PV system. They house the connections between solar modules and the cables that carry the electricity to the inverter. Junction boxes must be weatherproof and durable, protecting the electrical connections from moisture, dust, and other environmental hazards. In addition to protecting the system, well-designed junction boxes also facilitate easier maintenance and troubleshooting.
- Protection Devices: Protection devices, including fuses, circuit breakers, and surge protectors, are critical for ensuring the safety of the PV system. These components prevent electrical overloads, short circuits, and damage from lightning strikes or other surges. In grid-tied systems, protection devices also help maintain grid stability by preventing harmful feedback into the grid. Compliance with safety standards is particularly important in regions like Germany and Austria, where regulations are stringent and safety is a top priority.
4.2 Configuration Best Practices for BOS Components
Configuring BOS components correctly is key to optimizing the performance and durability of a PV system. Best practices for configuration involve not only selecting high-quality components but also ensuring that they are properly installed and maintained.
- Optimizing Mounting Structures: The configuration of mounting structures should take into account the specific conditions of the installation site. For rooftop installations, it’s important to consider the roof’s material, slope, and load-bearing capacity. In Germany and Austria, where snow load can be significant, mounting structures must be designed to withstand heavy snow accumulation without compromising the integrity of the roof or the solar modules. Ground-mounted systems, on the other hand, require careful consideration of soil conditions and anchoring methods to prevent shifting or settling over time.
- Ensuring Proper Wiring and Connections: The configuration of wiring and connections should minimize voltage drops and energy losses. This involves using the correct wire size for the distance between components and ensuring that all connections are secure and corrosion-resistant. In addition, wiring should be routed to minimize exposure to the elements, reducing the risk of damage from UV radiation, moisture, or pests. Regular inspection and maintenance of wiring are essential to detect and address any issues before they lead to system failure.
- Strategic Placement of Junction Boxes: Junction boxes should be strategically placed to minimize cable lengths and to protect the connections from environmental exposure. In rooftop installations, junction boxes are often placed under the modules or on the roof, where they are easily accessible but still protected from direct sunlight and precipitation. For ground-mounted systems, junction boxes can be housed in protective enclosures near the base of the mounting structure. The goal is to balance accessibility for maintenance with protection from environmental factors.
- Incorporating Comprehensive Protection Devices: The protection devices in a PV system should be configured to cover all potential points of failure. This includes installing fuses or circuit breakers at the array level, as well as surge protectors to guard against transient voltage spikes. In grid-tied systems, additional protective relays may be required to isolate the system from the grid in case of a fault. Ensuring that all protection devices are properly rated for the system’s voltage and current levels is critical to preventing damage and ensuring safety.
4.3 Real-World Applications: Germany and Austria
Germany and Austria have stringent regulations governing the installation and operation of PV systems, which emphasize the importance of high-quality BOS components and proper configuration. In these countries, the adoption of best practices for BOS configuration has led to the development of PV systems that are not only efficient but also highly durable and safe.
For example, in Germany, the combination of high-quality mounting structures and robust wiring practices has been instrumental in enabling rooftop PV installations to withstand extreme weather conditions, including heavy snow and strong winds. The country’s commitment to safety is also reflected in the widespread use of advanced protection devices, which are mandated by national regulations.
In Austria, the focus on optimizing BOS components is particularly evident in ground-mounted solar farms, where careful consideration is given to soil conditions and mounting techniques. The use of durable, weather-resistant junction boxes and comprehensive protection systems ensures that these large-scale installations maintain high levels of performance and reliability over their operational lifespan.
Both Germany and Austria serve as leading examples of how the careful selection and configuration of BOS components can significantly enhance the performance and longevity of PV systems, making them a model for other regions to follow.
5.System Design and Configuration: Optimizing Photovoltaic Installations
Designing and configuring a photovoltaic (PV) system is a complex process that requires careful consideration of various factors to ensure optimal performance, efficiency, and longevity. The success of a PV installation hinges on a well-thought-out design that takes into account site-specific conditions, energy requirements, and regulatory constraints. This section delves into the key principles of PV system design, strategies for different types of installations, and real-world case studies from Germany and Austria.
5.1 Design Principles for Photovoltaic Systems
The design of a PV system begins with a comprehensive assessment of the site where the system will be installed. This assessment includes evaluating the available space, orientation, shading, and local climate conditions. The goal is to maximize energy production while minimizing losses due to inefficiencies or environmental factors.
- Site Assessment and Shading Analysis: A thorough site assessment is the foundation of any successful PV system design. This involves measuring the available area for solar modules, determining the optimal orientation (typically south-facing in the northern hemisphere), and evaluating potential shading obstacles, such as trees, buildings, or other structures. Shading can significantly reduce the efficiency of a PV system, so it is crucial to conduct a shading analysis to identify and mitigate its impact. In Germany and Austria, where seasonal variations in sunlight are pronounced, careful attention to shading is essential for maintaining consistent energy production throughout the year.
- System Sizing and Energy Demand: Once the site assessment is complete, the next step is to size the PV system appropriately. This involves calculating the energy demand of the site and determining the number of solar modules and the capacity of the inverter needed to meet that demand. System sizing must balance the goal of maximizing energy production with the physical and financial constraints of the installation. In residential settings, the system is often sized to meet the household’s average daily consumption, while in commercial or industrial applications, the system may be scaled to offset a significant portion of the facility’s energy use.
- Component Selection: The choice of components, including solar modules, inverters, and energy storage systems, is critical to the overall performance of the PV system. High-efficiency modules can generate more electricity in a limited space, making them ideal for installations with space constraints. The inverter must be chosen to match the capacity of the PV array and should include features such as Maximum Power Point Tracking (MPPT) to optimize energy harvest. In grid-tied systems, it is also important to ensure that the inverter complies with local grid standards and regulations.
5.2 Configuration Strategies for Different Types of Installations
PV systems can be configured in various ways depending on the specific needs of the installation. The two primary types of PV system configurations are grid-tied and off-grid, each with its own set of advantages and challenges.
- Grid-Tied Systems: Grid-tied PV systems are connected to the public electricity grid, allowing excess energy produced by the system to be fed back into the grid. This configuration is the most common in Germany and Austria, where robust grid infrastructure and favorable policies support the integration of solar power. Grid-tied systems typically do not require large energy storage solutions, as the grid serves as a virtual battery, absorbing excess energy and providing power when solar production is low. Key considerations for grid-tied systems include ensuring grid compatibility, maximizing self-consumption, and navigating feed-in tariffs or net metering policies.
- Off-Grid Systems: Off-grid PV systems operate independently of the public grid, making them suitable for remote locations or applications where grid access is unreliable or unavailable. These systems require energy storage solutions, such as batteries, to provide power during periods when solar production is insufficient. Off-grid systems are often used in rural areas, cabins, or for specialized applications like telecommunications or agricultural operations. The design of off-grid systems must carefully balance the energy production of the PV array with the storage capacity to ensure a reliable power supply throughout the day and night. In Austria, off-grid systems are sometimes used in alpine regions where grid access is limited.
- Hybrid Systems: Hybrid PV systems combine elements of both grid-tied and off-grid configurations. These systems are connected to the grid but also include energy storage solutions to increase self-consumption and provide backup power during grid outages. Hybrid systems are becoming increasingly popular in Germany, where energy storage incentives and the desire for energy independence drive adoption. The design of hybrid systems must account for both the integration with the grid and the management of stored energy to optimize performance.
5.3 Tools and Software for PV System Design
The design of PV systems is greatly aided by specialized tools and software that allow for precise calculations and simulations. These tools help designers optimize system performance, predict energy yields, and ensure compliance with local regulations.
- Solar Design Software: Software like PV*SOL, PVSyst, and HelioScope are widely used in the industry to model PV systems. These tools allow designers to input site-specific data, including geographic location, shading analysis, and module specifications, to generate accurate predictions of system performance. They also provide insights into financial metrics, such as payback periods and return on investment, which are critical for project feasibility assessments.
- Shading Analysis Tools: Tools such as Solar Pathfinder and Solmetric SunEye are used to conduct detailed shading analyses. These tools provide visual representations of potential shading obstacles throughout the year, allowing designers to optimize module placement and orientation to minimize shading losses.
- Energy Modeling Software: For systems that include energy storage, software like HOMER Energy or SAM (System Advisor Model) can model the interaction between the PV array, storage, and the grid. These tools help designers size the storage system appropriately and optimize the configuration to meet the specific energy demands of the site.
5.4 Case Studies: Germany and Austria
Germany and Austria provide excellent examples of successful PV system designs that have been optimized for their specific environments and regulatory contexts. The following case studies highlight different approaches to system design and configuration in these countries.
- Residential Grid-Tied System in Germany: A typical residential PV system in Germany might involve a 5 kW array installed on a south-facing rooftop. The system is designed to maximize self-consumption, with any excess energy fed back into the grid under Germany’s feed-in tariff scheme. The design process involves careful consideration of shading from nearby trees and buildings, as well as the selection of high-efficiency modules and a smart inverter to ensure compliance with grid standards.
- Commercial Hybrid System in Austria: In Austria, a commercial facility might install a 100 kW hybrid PV system to reduce energy costs and increase resilience against grid outages. The system includes a lithium-ion battery storage solution capable of providing backup power for several hours. The design process focuses on integrating the storage system with the grid to optimize energy usage and take advantage of Austria’s incentives for energy storage. The system also includes a sophisticated monitoring platform that allows the facility manager to track performance and make adjustments in real-time.
- Off-Grid Alpine Installation in Austria: In a remote alpine region of Austria, an off-grid PV system is designed to power a mountain cabin. The system includes a 10 kW solar array and a battery storage system with sufficient capacity to supply energy during extended periods of low sunlight. The design takes into account the harsh environmental conditions, with robust mounting structures and weather-resistant BOS components. The system is configured to maximize energy independence, with careful attention to load management and energy conservation.
5.5 Regulatory Considerations and Incentives
Both Germany and Austria have well-developed regulatory frameworks and incentive programs that influence the design and configuration of PV systems. Understanding these regulations and incentives is crucial for ensuring that the system is compliant and that the project can take full advantage of available support.
- Feed-In Tariffs and Net Metering: In Germany, the Renewable Energy Sources Act (EEG) provides a stable framework for feed-in tariffs, which compensate PV system owners for the energy they feed into the grid. Similarly, Austria offers feed-in tariffs and net metering schemes that encourage the adoption of solar power. The design of grid-tied systems must account for these policies to maximize financial returns.
- Energy Storage Incentives: Both Germany and Austria offer incentives for energy storage systems, which are increasingly being integrated into PV installations. These incentives can significantly reduce the upfront cost of storage solutions and make hybrid or off-grid systems more economically viable.
- Building Codes and Grid Connection Standards: Compliance with local building codes and grid connection standards is essential for any PV system. In Germany and Austria, these regulations ensure that systems are safe, reliable, and compatible with the broader energy infrastructure. The design process must incorporate these standards to avoid delays and additional costs during installation.
6.Maintenance and Monitoring: Ensuring the Longevity and Efficiency of Photovoltaic Systems
Photovoltaic (PV) systems are a significant investment, and their long-term success depends on proper maintenance and effective monitoring. Regular maintenance ensures that the system operates efficiently, while monitoring allows for the early detection of issues, minimizing downtime and maximizing energy production. This section explores the importance of maintenance and monitoring, key tasks involved, and best practices, with a focus on applications in Germany and Austria.
6.1 The Importance of Maintenance in Photovoltaic Systems
Maintenance is crucial to the longevity and performance of a PV system. Over time, various factors such as dust accumulation, weathering, and mechanical wear can reduce the efficiency of solar modules and other components. Regular maintenance helps mitigate these effects, ensuring that the system continues to generate electricity at optimal levels.
- Preventing Performance Degradation: One of the primary reasons for regular maintenance is to prevent the performance degradation of solar modules. Dust, dirt, bird droppings, and other debris can accumulate on the surface of the modules, reducing their ability to capture sunlight. In regions like Germany and Austria, where weather conditions can vary significantly, regular cleaning of the modules is essential to maintain their efficiency.
- Extending System Lifespan: Regular maintenance not only keeps the system operating efficiently but also extends its lifespan. Components such as inverters, wiring, and mounting structures can experience wear and tear over time. By inspecting and maintaining these components, potential issues can be addressed before they lead to system failures or costly repairs.
- Ensuring Safety: Safety is another critical aspect of maintenance. Electrical faults, loose connections, or damaged components can pose significant safety risks, including the potential for fires or electric shocks. Regular inspections help identify and rectify these issues, ensuring the safe operation of the PV system.
6.2 Key Maintenance Tasks for Photovoltaic Systems
Effective maintenance of a PV system involves several key tasks that should be performed regularly to ensure optimal performance and safety.
- Cleaning Solar Modules: Cleaning the solar modules is one of the most important maintenance tasks. Depending on the location and environmental conditions, modules may need to be cleaned every few months or more frequently. In Germany and Austria, where seasonal changes can lead to varying levels of dust and debris, cleaning schedules should be adjusted accordingly. It is important to use the correct cleaning techniques and materials to avoid damaging the modules. For example, soft brushes and deionized water are recommended to prevent scratching the surface.
- Inspecting Electrical Connections: Regular inspection of electrical connections is essential to ensure that the system is operating safely and efficiently. Loose or corroded connections can lead to energy losses, overheating, or even fire hazards. During inspections, all connections should be checked for tightness, and any signs of corrosion should be addressed immediately. This task is particularly important for older systems, where connections may have degraded over time.
- Checking Inverters and Monitoring Systems: Inverters are the heart of the PV system, and their performance is critical to overall system efficiency. Regularly checking the inverter’s operation, including its display and any warning lights, can help identify potential issues early. Inverters typically have a lifespan of around 10 to 15 years, so regular checks are important as they age. Monitoring systems, which track the performance of the entire PV system, should also be regularly reviewed to ensure they are functioning correctly.
- Inspecting Mounting Structures: The mounting structures that support the solar modules must be inspected regularly for signs of wear, corrosion, or damage. In areas with harsh weather conditions, such as heavy snow or high winds, these inspections are particularly important. Any signs of structural weakness should be addressed promptly to prevent damage to the modules or the roof.
- Testing and Replacing Batteries: For systems that include energy storage, such as off-grid or hybrid systems, regular testing and maintenance of the batteries are crucial. Batteries have a limited lifespan and will gradually lose their capacity over time. Regular testing ensures that the batteries are still performing effectively, and replacements can be planned before the batteries fail completely. In Germany and Austria, where energy storage is becoming increasingly common, maintaining battery health is essential for system reliability.
6.3 The Role of Monitoring in Photovoltaic Systems
Monitoring plays a crucial role in maintaining the efficiency and reliability of a PV system. Advanced monitoring systems provide real-time data on the performance of the system, allowing for quick detection of issues and informed decision-making.
- Real-Time Performance Tracking: Modern PV systems are equipped with monitoring platforms that track various performance metrics in real-time, including energy production, system efficiency, and inverter status. This data is invaluable for identifying any deviations from expected performance, which could indicate underlying issues. In Germany and Austria, where feed-in tariffs and incentives are tied to energy production, monitoring systems ensure that the system is operating optimally to maximize financial returns.
- Remote Diagnostics and Troubleshooting: Many monitoring systems offer remote diagnostics capabilities, allowing system operators or maintenance providers to identify and address issues without the need for on-site visits. This is particularly beneficial for large-scale installations or systems in remote locations. By analyzing the data provided by the monitoring system, technicians can often pinpoint the cause of a problem and take corrective action quickly, minimizing downtime.
- Predictive Maintenance: Predictive maintenance uses data from monitoring systems to anticipate potential issues before they lead to system failures. By analyzing trends in system performance, such as a gradual decline in energy output or an increase in inverter temperature, maintenance can be scheduled proactively. This approach not only improves system reliability but also reduces maintenance costs by addressing issues before they escalate.
- Compliance and Reporting: In many regions, including Germany and Austria, PV systems are subject to regulatory requirements and performance standards. Monitoring systems can generate reports that demonstrate compliance with these regulations, ensuring that the system remains eligible for incentives and avoiding potential penalties. These reports can also be useful for system owners who want to track the return on investment and verify that the system is performing as expected.
6.4 Best Practices for Maintenance and Monitoring
To ensure the long-term success of a PV system, it is important to follow best practices for maintenance and monitoring. These practices help optimize system performance, reduce the risk of failures, and extend the system’s lifespan.
- Establish a Regular Maintenance Schedule: Developing and adhering to a regular maintenance schedule is crucial for keeping the PV system in optimal condition. This schedule should be based on the specific needs of the system, taking into account factors such as the local environment, system size, and component lifespans. In regions like Germany and Austria, where weather conditions can vary throughout the year, the maintenance schedule may need to be adjusted seasonally.
- Use Qualified Professionals: While some maintenance tasks, such as cleaning modules, can be performed by the system owner, more complex tasks should be handled by qualified professionals. This includes inspecting electrical connections, checking inverters, and performing battery maintenance. Hiring professionals ensures that the work is done safely and correctly, reducing the risk of damage to the system.
- Leverage Monitoring Data for Decision-Making: Monitoring systems provide a wealth of data that can be used to make informed decisions about maintenance, system upgrades, and energy management. System owners and operators should regularly review this data and use it to identify trends, optimize performance, and plan for future needs. In Germany and Austria, where energy policies and incentives are closely tied to system performance, effective use of monitoring data can have significant financial benefits.
- Stay Informed About Technological Advances: The field of PV technology is continuously evolving, with new advancements in both hardware and software. Staying informed about these developments can help system owners and operators take advantage of new technologies that improve efficiency, reduce maintenance costs, or enhance system reliability. This is particularly important in dynamic markets like Germany and Austria, where technological innovation plays a key role in the energy transition.
6.5 Real-World Applications: Germany and Austria
Germany and Austria are known for their advanced PV systems and comprehensive maintenance and monitoring practices. These countries have set high standards for system performance and safety, and their approaches to maintenance and monitoring serve as models for other regions.
- German Residential Systems: In Germany, residential PV systems often include sophisticated monitoring platforms that allow homeowners to track their energy production and consumption in real-time. These systems are connected to smart home devices, enabling automated adjustments to maximize self-consumption and reduce energy costs. Maintenance is typically scheduled based on the monitoring data, ensuring that the system remains in top condition year-round.
- Austrian Commercial Installations: Commercial PV installations in Austria frequently use predictive maintenance strategies to enhance system reliability and reduce operational costs. For example, a large solar farm in Austria might use monitoring data to anticipate inverter failures and replace components before they fail, avoiding costly downtime. These systems are also designed to comply with Austria’s strict energy regulations, with regular reports generated to demonstrate ongoing compliance.
- Alpine PV Systems in Austria: In the challenging environment of the Austrian Alps, off-grid PV systems require meticulous maintenance and monitoring to ensure reliable operation. These systems often power remote cabins or weather stations, where access is difficult, and reliability is critical. Advanced monitoring systems provide real-time data that can be accessed remotely, allowing operators to manage the system without needing to visit the site frequently. Maintenance tasks, such as clearing snow from modules and inspecting batteries, are scheduled based on environmental conditions and system performance data.
7.Future Trends and Innovations: Shaping the Next Generation of Photovoltaic Systems
As the global energy landscape continues to evolve, so too does the technology that powers photovoltaic (PV) systems. Innovations in materials, system design, and digital integration are driving the next generation of solar power, making it more efficient, cost-effective, and versatile than ever before. This section explores some of the most promising future trends and innovations in PV technology, focusing on advancements in solar cells, system integration, and the growing role of artificial intelligence (AI) and the Internet of Things (IoT). Additionally, it examines how these innovations are being adopted in Germany and Austria, two countries at the forefront of the renewable energy transition.
7.1 Emerging Technologies in Solar Cells
One of the most exciting areas of innovation in PV systems is the development of new solar cell technologies. These advancements aim to improve the efficiency, durability, and versatility of solar modules, making solar energy more accessible and competitive.
- Perovskite Solar Cells: Perovskite solar cells have generated significant interest in the renewable energy sector due to their potential for high efficiency and low production costs. Unlike traditional silicon-based cells, perovskite cells can be manufactured using simpler, less expensive processes. They also have the potential to achieve higher efficiencies by absorbing a broader spectrum of sunlight. However, challenges remain in terms of long-term stability and scalability. Researchers in Germany are actively working on overcoming these hurdles, with the aim of commercializing perovskite solar cells in the near future.
- Bifacial Solar Cells: Bifacial solar cells are designed to capture sunlight on both sides of the module, increasing the overall energy yield. This is particularly beneficial in environments with high albedo surfaces, such as snow-covered or reflective ground, which can reflect additional light onto the backside of the panels. In countries like Austria, where snow is common in winter, bifacial modules can significantly boost energy production. As the cost of bifacial technology decreases, it is expected to become more widely adopted in both residential and commercial installations.
- Building-Integrated Photovoltaics (BIPV): BIPV represents a convergence of solar technology and building design, where solar cells are integrated directly into building materials such as windows, façades, and roofing. This approach allows buildings to generate their own electricity while maintaining aesthetic appeal. In Europe, where regulations often encourage or mandate energy-efficient building practices, BIPV is gaining traction. In Germany, for example, BIPV is being used in new construction projects to meet strict energy performance standards. As the technology matures, it is expected to play a significant role in urban solar energy generation.
7.2 System Integration and Smart Energy Solutions
As PV systems become more advanced, the integration of solar power with other energy systems and smart technologies is becoming increasingly important. This trend is driving the development of more flexible, intelligent, and resilient energy systems.
- Hybrid Systems with Advanced Energy Storage: Hybrid PV systems, which combine solar power with energy storage, are becoming more sophisticated. Advances in battery technology, particularly lithium-ion and emerging solid-state batteries, are making it easier to store excess solar energy for use during periods of low sunlight or peak demand. These systems are especially valuable in regions with variable weather patterns or high electricity costs. In Germany and Austria, the adoption of hybrid systems is being driven by incentives for energy storage and the desire for greater energy independence. Future developments are likely to focus on improving battery efficiency, reducing costs, and integrating storage more seamlessly with PV systems.
- Smart Inverters and Grid Interaction: Smart inverters are a critical component of modern PV systems, enabling better interaction with the electrical grid. These inverters can perform advanced functions such as voltage regulation, reactive power control, and grid frequency stabilization. In Germany, where the grid is increasingly powered by renewable energy, smart inverters help maintain grid stability despite the intermittent nature of solar power. As more PV systems are connected to the grid, the role of smart inverters will continue to grow, facilitating the integration of large amounts of solar energy without compromising grid reliability.
- Energy Management Systems: Energy management systems (EMS) are becoming a key feature of PV installations, particularly in commercial and industrial settings. These systems use sophisticated algorithms to optimize energy production, consumption, and storage based on real-time data. EMS can manage multiple energy sources, including solar, wind, and grid power, to ensure that energy is used as efficiently as possible. In Austria, EMS is being used to optimize energy use in factories and large commercial buildings, helping to reduce energy costs and improve sustainability. The future of EMS lies in its ability to integrate with AI and IoT technologies, creating fully autonomous energy systems that can adapt to changing conditions without human intervention.
7.3 The Role of Artificial Intelligence and IoT in PV Systems
Artificial intelligence (AI) and the Internet of Things (IoT) are poised to revolutionize the way PV systems are designed, operated, and maintained. These technologies offer new opportunities for optimizing performance, reducing costs, and enhancing system reliability.
- AI-Driven System Optimization: AI can be used to analyze vast amounts of data from PV systems, identifying patterns and making adjustments to optimize performance. For example, AI algorithms can predict periods of low solar production based on weather forecasts and adjust the operation of the system accordingly, such as by discharging stored energy in anticipation of cloudy days. AI can also be used to detect anomalies in system performance, enabling predictive maintenance that reduces downtime and repair costs. In Germany, researchers are exploring the use of AI to enhance the efficiency of large-scale solar farms, where even small improvements can result in significant energy gains.
- IoT-Enabled Monitoring and Control: IoT devices are increasingly being integrated into PV systems, providing real-time monitoring and control capabilities. These devices can communicate with each other and with centralized management systems, allowing for more precise control of energy production and consumption. For example, IoT sensors can monitor the temperature, humidity, and sunlight levels at a PV installation, automatically adjusting the tilt angle of the panels or the operation of cooling systems to maximize efficiency. In Austria, IoT technology is being used in smart homes to manage energy use, integrating PV systems with home automation systems for seamless control of energy flows.
- Blockchain for Energy Transactions: Blockchain technology, best known for its role in cryptocurrencies, is also finding applications in the energy sector. In the context of PV systems, blockchain can be used to facilitate peer-to-peer energy trading, where excess solar energy produced by one system can be sold directly to another user. This decentralized approach to energy distribution could enable more efficient use of renewable energy and reduce reliance on traditional utilities. Germany has been a leader in exploring blockchain for energy, with several pilot projects underway that allow households with PV systems to trade energy with their neighbors.
7.4 Adoption and Impact in Germany and Austria
Germany and Austria are at the forefront of adopting and implementing these future trends and innovations in PV technology. Both countries have strong commitments to renewable energy, supported by robust regulatory frameworks and financial incentives.
- Germany: Germany’s Energiewende, or energy transition, has made the country a global leader in renewable energy adoption. The integration of smart technology, AI, and IoT into PV systems is a natural extension of this transition. Germany’s focus on grid stability, driven by the high penetration of solar power, has accelerated the adoption of smart inverters and energy management systems. The country is also a hub for research and development in solar technology, with numerous projects exploring the potential of perovskite cells, BIPV, and blockchain for energy trading.
- Austria: Austria’s renewable energy strategy emphasizes energy independence and sustainability, with a strong focus on solar power. The adoption of hybrid systems and energy storage is growing rapidly, supported by government incentives and public interest in reducing reliance on fossil fuels. Austria is also a leader in smart home technology, integrating PV systems with IoT-enabled energy management systems to create more sustainable living environments. The country’s mountainous terrain and varying climate conditions make it an ideal testing ground for advanced solar technologies, such as bifacial modules and AI-driven optimization.
7.5 Challenges and Opportunities
While the future of PV systems is bright, several challenges must be addressed to fully realize the potential of these innovations.
- Cost and Scalability: Many of the emerging technologies, such as perovskite solar cells and advanced energy storage, are still in the early stages of development and are not yet cost-competitive with existing solutions. Scaling these technologies to meet global demand will require continued research, investment, and support from both the public and private sectors.
- Regulatory Hurdles: As PV systems become more integrated with digital technologies, regulatory frameworks will need to evolve to address new challenges, such as data privacy, cybersecurity, and the safe operation of AI-driven systems. Policymakers in Germany and Austria are already working to update regulations, but ongoing dialogue between industry and government will be essential.
- Public Acceptance and Education: The widespread adoption of new technologies often depends on public acceptance and understanding. As AI, IoT, and blockchain become more prevalent in the energy sector, efforts to educate the public about their benefits and address concerns will be important for ensuring their successful integration.
Despite these challenges, the opportunities for innovation in PV systems are vast. By embracing these trends, Germany and Austria are well-positioned to lead the world in the next phase of the renewable energy revolution.
8.Conclusion: The Future of Photovoltaic Systems
Photovoltaic (PV) systems have emerged as a cornerstone of the global transition to renewable energy. As the world moves toward reducing its reliance on fossil fuels, the importance of solar energy cannot be overstated. This guide has provided an in-depth exploration of the components and configurations that make up PV systems, highlighting the importance of each element in ensuring optimal performance and sustainability. In this final section, we will summarize the key points discussed throughout the article, reflect on the future of PV technology, and underscore the significance of continued innovation and adoption, particularly in leading markets like Germany and Austria.
8.1 Recap of Key Points
Throughout this guide, we have explored the essential components and configurations of PV systems, each playing a critical role in the overall effectiveness and efficiency of solar power generation:
- Solar Modules: The heart of any PV system, solar modules are responsible for converting sunlight into electricity. We discussed the different types of solar modules, such as monocrystalline, polycrystalline, and thin-film, and examined their respective efficiencies, costs, and applications. Innovations like perovskite and bifacial solar cells promise to further enhance the capabilities of these modules, making solar energy even more accessible and efficient.
- Inverters: Inverters are crucial for converting the direct current (DC) generated by solar modules into alternating current (AC), which can be used by most electrical devices and fed into the grid. We covered the various types of inverters, including string, central, and microinverters, as well as the importance of key specifications like efficiency and maximum power point tracking (MPPT). The rise of smart inverters is particularly noteworthy, offering advanced grid-support functions that are increasingly necessary as solar penetration grows.
- Energy Storage Solutions: Energy storage is essential for balancing the intermittent nature of solar power, enabling PV systems to provide reliable energy even when the sun isn’t shining. We explored the different types of storage systems, including lead-acid, lithium-ion, and flow batteries, and discussed their applications in residential, commercial, and industrial settings. The integration of energy storage with PV systems, particularly in hybrid configurations, is becoming more common, driven by declining battery costs and increasing demand for energy independence.
- Balance of System (BOS) Components: Often overlooked, BOS components such as mounting structures, wiring, junction boxes, and protection devices are vital for the safe and efficient operation of PV systems. Proper configuration of these components ensures the long-term durability and performance of the system, reducing the risk of failures and optimizing energy output.
- System Design and Configuration: Effective system design and configuration are key to maximizing the benefits of PV installations. We discussed the importance of site assessment, system sizing, and component selection, as well as strategies for different types of installations, including grid-tied, off-grid, and hybrid systems. Real-world examples from Germany and Austria illustrated how these principles are applied in practice, leading to successful, efficient PV installations.
- Maintenance and Monitoring: Regular maintenance and ongoing monitoring are essential to keeping PV systems operating at peak efficiency. We outlined key maintenance tasks, such as cleaning solar modules, inspecting electrical connections, and testing batteries, as well as the role of monitoring systems in tracking performance and identifying issues early. In regions like Germany and Austria, where regulatory standards are high and performance incentives are common, these practices are particularly important.
- Future Trends and Innovations: The future of PV systems is bright, with numerous innovations on the horizon that promise to enhance the efficiency, reliability, and versatility of solar energy. Emerging technologies such as perovskite solar cells, AI-driven system optimization, and IoT-enabled monitoring are set to revolutionize the industry. Germany and Austria are at the forefront of adopting these innovations, positioning themselves as leaders in the global transition to renewable energy.
8.2 Reflections on the Future of PV Technology
As PV technology continues to evolve, it is clear that the future holds great promise for solar energy. The ongoing development of more efficient solar cells, advanced inverters, and integrated energy storage solutions will make PV systems more accessible and cost-effective for a broader range of applications. In particular, the integration of digital technologies like AI and IoT into PV systems is expected to drive significant improvements in system performance, reliability, and user engagement.
In addition to technological advancements, the continued growth of the PV market will depend on supportive regulatory environments and financial incentives. Countries like Germany and Austria have shown that strong government policies can accelerate the adoption of renewable energy, creating stable markets for PV systems and encouraging innovation. As other countries look to replicate these successes, the global PV market is likely to expand, driving further investment and development in solar technology.
8.3 The Role of Innovation and Adoption
Innovation and adoption are two sides of the same coin when it comes to the future of PV systems. On the one hand, ongoing research and development are critical for pushing the boundaries of what solar technology can achieve. On the other hand, widespread adoption of these innovations is necessary to realize their full potential and drive down costs through economies of scale.
Germany and Austria serve as prime examples of how innovation and adoption can work together to advance the renewable energy transition. By fostering a culture of innovation, supporting R&D efforts, and creating favorable conditions for the deployment of new technologies, these countries have positioned themselves at the cutting edge of the global PV market. Their experiences provide valuable lessons for other regions seeking to accelerate their own transitions to renewable energy.
8.4 Final Thoughts: Embracing the Solar Future
The journey toward a more sustainable energy future is well underway, and photovoltaic systems are playing a central role in this transformation. As this guide has shown, the components and configurations of PV systems are diverse, complex, and continually evolving. Understanding these elements is essential for anyone involved in the design, installation, or management of solar power systems.
As we look to the future, it is clear that solar energy will continue to grow in importance, driven by ongoing technological advancements, supportive policies, and increasing public demand for clean, renewable energy. Whether you are a seasoned engineer, a technical manager, or a decision-maker in the energy sector, staying informed about the latest developments in PV technology is crucial to making the most of the opportunities that lie ahead.
Germany and Austria are leading the way, demonstrating how solar energy can be integrated into the fabric of society, providing reliable, sustainable power for generations to come. By embracing the innovations and best practices outlined in this guide, other regions can follow in their footsteps, contributing to a cleaner, more sustainable world.