This review explores the effect of scaffold technology and nanomaterials on antibody biosensors’ performance. These innovations increase the sensitivity of biosensors for rapidly identifying bacterial pathogens in healthcare and environmental safety. The article discusses the combination of new materials and designs in creating these biosensors, their challenges, and their potential. It compares traditional and advanced biosensors, highlights the use of nanotechnology, and looks at different detection methods, such as electrochemical and optical techniques, to recognize bacteria. Furthermore, this review emphasizes the importance of developing future antibody biosensors for diagnostics and treatment.
Author ID: 2024024866
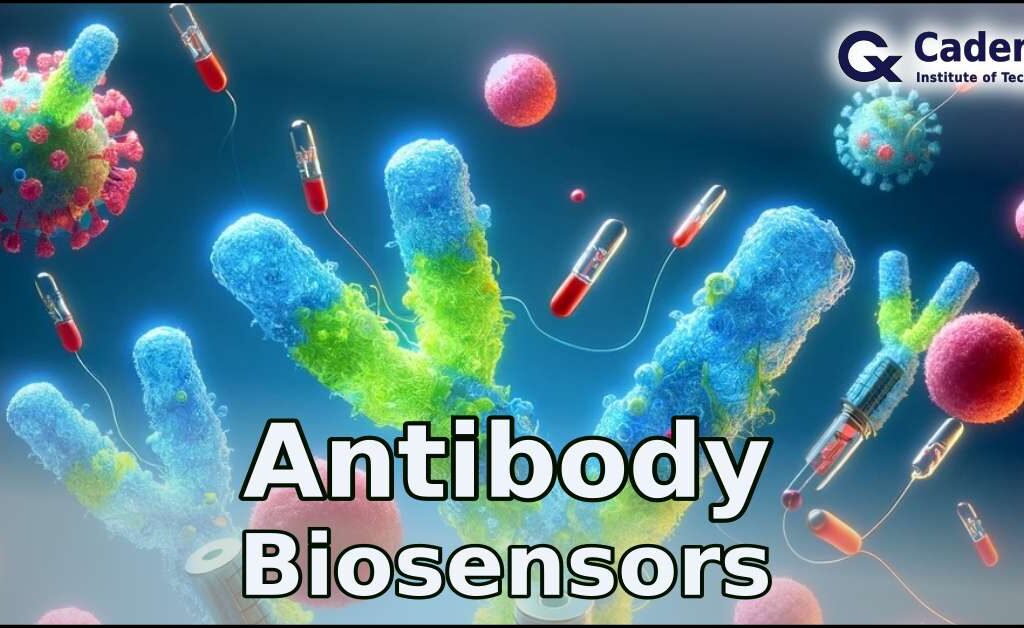
1. Introduction
Rapid detection of pathogenic bacteria is critical in healthcare and environmental safety. Traditional detection methods, including culture-based assays and microscopy, are slow and complex, causing delays. However, biosensors, incorporating biological elements like aptamers, DNA, and antibodies, provide a faster alternative. Moreover, biosensors include transducers to convert biological mechanisms into chemical signals and detectors, such as electrochemical and optical systems, for measuring signals. This speed is vital for early healthcare diagnosis and treatment, improving patient outcomes and disease management. Similarly, biosensors swiftly identify bacterial contamination in environmental safety, preventing ecological harm and safeguarding public health. Their high sensitivity and specificity surpass traditional methods, making them more effective in monitoring and controlling bacterial threats.
Nowadays, antibody biosensors are more popular for detecting biological targets, especially pathogenic bacteria. These biosensors leverage the specificity of antibody-antigen interactions to convert biological recognition events into measurable electrical signals. Their core functionality is transducing these interactions into quantifiable signals, enabling the sensitive and specific detection and quantification of pathogens. The principle of these biosensors is the immobilization of antibodies on an electrode surface. The binding between antibodies and a target antigen, like a specific pathogen, alters the electrode’s electrical properties. This change, measured as current, potential, or charge transfer resistance variations, directly correlates to the antigen’s concentration in the sample.
The main part of antiboy biosensors is the electrode material, which must facilitate efficient electron transfer while maintaining stability and biocompatibility. This objective can be achieved by applying nanotechnology and utilizing scaffolding techniques. Advances in nanotechnology propelled the development of novel electrode materials, enhancing sensitivity and enabling the miniaturization of biosensors. Nanomaterials, such as carbon nanotubes and graphene, have been extensively used due to their high surface area, electrical conductivity, and ability to promote electron transfer reactions.
Furthermore, using scaffolding strategies is another factor in enhancing the sensitivity of electrochemical antibodies. This approach involves designing and incorporating structural frameworks or scaffolds within the biosensor system to optimize antibody immobilization and orientation. It creates a stable antibody environment, improving their interaction with target antigens and amplifying sensor sensitivity. This technique enhances biosensor performance and reliability in detecting trace molecules, making them essential in biomedicine.
This review represents antibody biosensors, highlighting their crucial role in swiftly and accurately detecting pathogenic bacteria, a feature that makes them indispensable in healthcare diagnostics, environmental monitoring, and biodefense sectors. Furthermore, the article examines the current limitations of these biosensors and explores potential strategies researchers might employ to boost the performance of these point-of-care devices in future applications.
2. Scaffold Methods
In the cutting-edge field of biosensors, scaffolds emerge as vital elements that significantly enhance the detection mechanism, akin to the structural framework that supports a building. These scaffolds are essential for precisely identifying viruses and other biomarkers, providing a robust foundation for bio-recognition elements. The role of scaffolds extends beyond mere structural components, as they boost the sensor’s performance and sensitivity, marking a significant leap in biosensor technology.
The innovation in scaffold design has been pivotal in advancing biosensor capabilities, optimizing the interaction between biosensors and target molecules for more effective and faster detection. This design evolution enhances the effectiveness of biosensors, which is crucial for prompt medical intervention. Therefore, the meticulous arrangement and choice of scaffold materials have become key areas of research and development, aiming to maximize the efficiency and functionality of biosensors.
However, incorporating scaffolds into biosensors introduces particular challenges alongside the advantages. The complexity of fabrication and the need for precise calibration pose potential hurdles, reflecting the sophisticated nature of scaffold-enhanced biosensor development. Despite these challenges, the benefits, including improved sensitivity and rapid detection, highlight the transformative potential of scaffolds in the biosensor field.
Real-world case studies underscore the effectiveness of scaffold technology in boosting biosensor sensitivity, with applications demonstrating the capability of these advanced devices to detect diseases at earlier stages than previously possible. This breakthrough in early disease detection offers significant advantages in managing and controlling infectious diseases, showcasing the pivotal role of scaffold-enhanced biosensors in public health and diagnostics.
2.1. Comparison Traditional Methods and Scaffold-Enhanced Biosensors
Comparing traditional biosensors with those enhanced by scaffold technology reveals a stark difference in performance. Scaffold-enhanced biosensors boast greater sensitivity and specificity, making them far more reliable for early detection of diseases. This comparison underscores the transformative impact of scaffold methods in diagnostics, offering a more nuanced and effective approach to identify pathogens.
As is shown in Table. 1, the scaffold methods section encapsulates the progress and challenges in integrating scaffold technology with biosensors. Incorporating scaffolds, particularly those utilizing nanomaterials and innovative structural designs, marks a revolutionary step in enhancing the detection capabilities of biosensors. Despite the complexities involved in their development, the advantages of scaffold-enhanced biosensors — from increased sensitivity and faster results to potential for early disease detection — underscore their critical contribution to modern diagnostics and their vast potential for the future of public health.
Table 1. Comparing scaffold-enhanced biosensors with conventional techniques
Criteria | ELISA | Antigen Test | RT-PCR | IFA | Western Blot | Scaffold Methods |
---|---|---|---|---|---|---|
Sensitivity | High | Moderate | Very High | High | High | Enhanced |
Specificity | High | High | High | High | High | Higher |
Speed of Detection | Slow | Rapid | Rapid | Slow | Time-consuming | Faster |
Cost | Moderate | Low | High | High | Moderate to High | Potentially Lower |
Ease of Use | Trained Personnel | User-friendly | Specialized Equipment | Specialized Training | Technical Expertise | More User-Friendly |
Portability | Limited | Highly Portable | Limited | Limited | Not Portable | Enhanced Portability |
3. Nanomaterials and Innovative Structures in Antibody Bioensor
In the field of antibody biosensors, integrating nanomaterials and innovative structural designs has ushered in a new era of enhanced detection capabilities. Nanotechnology revolutionized the way these biosensors operate. It involves the use of nanoscale materials and structures, such as nanoparticles, nanowires, and nanotubes, which offer several advantages. Firstly, nanomaterials provide an enlarged surface area, allowing for greater antibody immobilization and thus improving sensitivity. Secondly, their unique physicochemical properties can enhance signal transduction mechanisms, making detection more efficient and reliable. Moreover, nanomaterials can synergize effectively with antibody receptors, facilitating the binding of target molecules with exceptional specificity.
Recently, innovative scaffold structures with nanomaterials resolve the boundaries of traditional biosensor designs. Beyond the conventional approaches, these structures optimize the arrangement of nanomaterials and antibodies, resulting in improved recognition and capture of target molecules. The role of surface area and reactivity in these scaffolds is paramount, as it directly influences the biosensor’s performance. These innovative designs significantly enhance sensitivity and accuracy by maximizing the surface area available for antibody immobilization and ensuring high reactivity.
Here, we discussed the transformative impact of nanotechnology and creative structural designs in improving the detection capabilities of these biosensors. Through increased surface area, efficient signal transduction, and optimized scaffold structures, antibody biosensors are becoming even more powerful tools for precise and reliable detection in various applications.
4. Detection methods of antibody biosensor
Antibody biosensors stand out in biomedical analysis for their efficient and sensitive detection of specific antigens, using antibody receptors to measure electrochemical signals. These biosensors are highly sensitive, cost-effective, and portable, making them ideal for diverse applications like disease diagnosis, environmental monitoring, and food safety. While they offer significant advantages, challenges include the need for frequent calibration and potential interference from other substances in samples. Nonetheless, their practicality in various fields makes them a valuable method in the healthcare system. To investigate the performance of biosensors, various approaches are employed, such as electrochemical and optical approaches.
4.1. Electrochemical approach
Electrochemical approaches encompass diverse techniques that play a pivotal role in biosensor technology. These methods include amperometry, potentiostat/galvanostat, impedance spectroscopy, cyclic voltammetry, Field-Effect Transistor (FET), Surface Plasmon Resonance (SPR), and Electrochemiluminescence (ECL). These various electrochemical approaches offer versatility and can be tailored to specific biosensing needs, making them essential tools in biosensor development and optimization.
4.1.1. Amperometry
Amperometric detection is a key analytical technique in electrochemistry designed to measure the current generated by the oxidation or reduction of an analyte under a constant potential applied to the working electrode. This method excels in sensitivity and selectivity, allowing the detection of low analyte levels. Its advantages include a wide linear response range, rapid response times, and compatibility with microfabricated devices, making it invaluable for creating portable analytical instruments. However, it faces challenges such as potential interference from other substances in the sample that can undergo similar electrochemical reactions, electrode fouling from reaction products, and the limitation that only electroactive substances can be detected.
4.1.2. Voltammetry
Voltammetric detection in antibody biosensors uses voltage sweeps to detect and measure specific antigens by their interaction with immobilized antibodies. This method is highly sensitive and specific, capable of detecting low biomarker concentrations and providing crucial quantitative data for early disease diagnosis. Voltammetric biosensors are also portable, making them ideal for on-the-spot testing. However, a limitation is that antigens need to be electroactive or modified to be so, adding complexity to assay design. Additionally, the technique might encounter interference from other substances in complex samples, potentially impacting measurement accuracy. Despite these challenges, voltammetric detection remains a key technique for quickly and precisely analyzing biological samples, with wide-reaching implications for healthcare and diagnostics.
4.1.3. Electrochemical Impedance spectroscopy
Impedimetric detection in antibody biosensors measures electrical impedance changes when antigens bind to antibodies on an electrode, offering label-free detection. This simple and sensitive technique allows detecting tiny antigen amounts without extra labeling or preparation. It’s also capable of real-time monitoring of molecular interactions. However, the accuracy of impedimetric detection can be affected by the sample’s ionic strength and composition, requiring precise testing conditions. Additionally, analyzing impedance changes accurately may need advanced instrumentation and data analysis. Despite these challenges, impedimetric detection is valued for its quick, sensitive, and straightforward analysis in various biomedical fields.
4.1.4. Field-Effect Transistor (FET)
Field-Effect Transistor (FET)-based antibody biosensors use FET devices’ sensitivity to electrical changes at their surfaces for detecting antigen-antibody interactions. This approach stands out for its high sensitivity and precision, capable of accurately identifying biomarkers at low concentrations. FET biosensors deliver real-time feedback, essential for quick diagnostics and ongoing monitoring, and their design supports miniaturization, making them ideal for portable, point-of-care devices. However, they can be affected by environmental factors like pH and ionic strength, impacting sensor stability and accuracy. Additionally, producing FET biosensors involves complex and expensive processes. Despite these hurdles, FET-based biosensors remain invaluable in biosensing, offering rapid and accurate detection of various biological targets.
4.1.5. Electrochemiluminescence (ECL)
Electrochemiluminescence (ECL) in antibody biosensors merges electrochemical techniques with luminescence to pinpoint specific antigen-antibody interactions, known for its exceptional sensitivity and precision, even detecting tiny amounts of biomarkers. ECL stands out for its strong signal boost, making it possible to identify target molecules in complex samples, and its adaptability across various fields, from healthcare diagnostics to tracking environmental changes. Despite its benefits, ECL’s complexity, the need for special reagents and equipment, and the demand for exact electrochemical condition control can drive up costs and present challenges. Yet, ECL’s unique blend of specific detection and sensitive luminescent measurement is vital in biosensing, ensuring reliable and thorough detection outcomes.
4.2. Optical Methode
Optical antibody biosensors are a crucial tool in diagnostics and research, offering high precision and rapid detection of biomarkers using antibodies to specifically target molecules. Their main advantage is their exceptional sensitivity and specificity, beneficial in clinical diagnostics, environmental monitoring, and pharmaceutical development. However, they face challenges such as high costs, the need for specialized equipment and trained personnel, and potential accuracy issues in complex samples. Despite these drawbacks, the accuracy and versatility of optical antibody biosensors make them invaluable in advancing healthcare and research.
4.2.1. Surface Plasmon Resonance (SPR)
Surface Plasmon Resonance (SPR) is a key technique in antibody biosensors, enabling the study of how antibodies and antigens bind together without labels. It’s valued for providing immediate, detailed data on how strongly and quickly these molecules interact and in what amounts. The great thing about SPR is that it doesn’t alter the biomolecules, thanks to its label-free detection, making test setup easier and keeping the samples natural. However, the high cost of SPR equipment and the need for expert handling are downsides, as its sensitivity to sensor surface quality and potential issues with unrelated molecules sticking. Yet, for creating and evaluating antibody treatments and diagnostics, SPR’s precise detection capabilities are crucial.
4.2.2. Fluorescence approach
This approach involves labeling antibodies or antigens with fluorescent tags. The presence of the target antigen leads to a fluorescent signal, which can be quantitatively measured. Techniques like fluorescence resonance energy transfer (FRET) and time-resolved fluorescence (TRF) fall under this category, offering high sensitivity and the ability to multiplex.
4.2.3. Colorimetric
Colorimetric biosensors use antibody-antigen interactions that result in a visible color change, which can be observed with the naked eye or measured spectrophotometrically. This method is straightforward and does not require sophisticated instruments, making it suitable for point-of-care applications.
4.2.4. Surface Enhanced Raman Scattering (SERS)
SERS enhances the Raman scattering signal of molecules adsorbed on rough metal surfaces or nanoparticles. When antibodies on these surfaces bind to their target antigens, the resulting SERS signal can be detected and analyzed. This technique offers high sensitivity and the capability to provide detailed information about the molecular structure.
4.2.5. Photonic Crystal Biosensors
These biosensors use a periodic optical nanostructure that affects the motion of photons. The binding of antigens to antibodies attached to the photonic crystal surface causes a change in the optical properties of the crystal, which can be measured to detect the presence of the target antigen. This method allows for label-free detection and can be engineered for high-throughput screening.
4.3. Comparision between electrochemical and optical techniques
Electrochemical methods like amperometry, voltammetry, etc. offer sensitivity and cost-efficiency for antibody biosensors, though they require calibration and can be sample-sensitive. Optical techniques, such as SPR and fluorescence, enable precise, label-free detection and real-time analysis but can be costly and environmentally sensitive. The choice between electrochemical and optical depends on the test’s sensitivity, specificity needs, and practical considerations Table 2.
Table 2. Comparison between electrochemical and optical techniques
Method | Type | Advantages | Disadvantages |
---|---|---|---|
Amperometry | Electrochemical | High sensitivity, rapid, compatible with microdevices | Potential interference, electrode fouling |
Voltammetry | Electrochemical | Exceptional sensitivity, compact design | Requires electroactive antigens, sample interference |
Electrochemical Impedance Spectroscopy | Electrochemical | Label-free, real-time monitoring | Influenced by ionic strength, needs sophisticated instruments |
Field-Effect Transistor (FET) | Electrochemical | High sensitivity, real-time, miniaturizable | Environmental sensitivity, complex fabrication |
Electrochemiluminescence (ECL) | Electrochemical | Strong signal amplification, versatile | Complexity, specialized equipment needed |
Surface Plasmon Resonance (SPR) | Optical | Real-time data, label-free | Expensive, sensitive to surface quality |
Fluorescence | Optical | High sensitivity, multiplexing | Needs special equipment, accuracy issues in complex samples |
Colorimetric | Optical | Simple, point-of-care suitable | Limited quantification, non-specific reactions possible |
Surface Enhanced Raman Scattering (SERS) | Optical | Detailed structure information, high sensitivity | Requires specific surfaces, complex interpretation |
Photonic Crystal Biosensors | Optical | Label-free, high-throughput | Complex fabrication, may need sophisticated equipment |
5. Applications of antibody biosensors in point-of-care devices
Bioauxilium Researchers utilized cutting-edge technology to measure cellular signals like ERK1/2, p38αβγ, and STAT3 by detecting phosphorylation levels. This method uses two antibodies: one attached to a Europium chelate (Eu-Ab1) and the other to a far-red fluorophore (FR-Ab2). Exciting the Europium chelate at 320 or 340 nm induces energy transfer to the far-red fluorophore, but only when the target protein is phosphorylated, emitting a unique signal at 665 nm. The signal’s intensity reflects the protein phosphorylation level, offering insights into cellular activities crucial for understanding health and disease. This assay significantly improves the accuracy of monitoring protein modifications, aiding in comprehending biological complexities [1].
Mikuła et al. designed a highly sensitive electrochemical biosensor for detecting anti-H1N1 antibodies in vaccinated mice sera. It employs a redox-active monolayer, offering a novel approach to immune response monitoring. The biosensor’s advantage lies in its sensitivity and specificity, enabling precise antibody detection. However, creating and calibrating the redox-active monolayer can be complex, potentially limiting accessibility and requiring specialized equipment and expertise. Despite this, the biosensor holds promise for improving influenza virus research and vaccine studies, offering valuable applications in healthcare and research [2].
Mahshid et al. present a novel approach to detecting HIV antibodies in a one-step process. The researchers utilize a peptide-mediated electrochemical steric hindrance assay, demonstrating its potential for efficient and rapid HIV antibody detection. This innovative method offers advantages in terms of simplicity and speed, potentially revolutionizing HIV testing. However, further validation and optimization may be required before its widespread adoption. The study marks a significant step forward in HIV diagnostics, showing promise for improved early detection and monitoring [3].
Creative Biolabs offers comprehensive antibody analysis services in the biopharmaceutical industry. Monoclonal antibodies (mAbs) are pivotal in biologics drug development, given their exceptional specificity, particularly in oncology, immunology, and anti-infective therapeutic areas. However, antibody product complexity poses unique development and manufacturing challenges. Creative Biolabs addresses these with services encompassing functions like antibody sequencing, affinity measurement, epitope binning, immunogenicity assessment, and advanced techniques like isothermal titration calorimetry (ITC), differential scanning calorimetry (DSC), and dual-polarization interferometry (DPI). With a seasoned academic team and decades of antibody research experience, they designed antibody therapeutic development [4].
6. Limitations of diagnostic antibody biosensors
Antibody biosensors are amazing tools for detecting diseases and pollutants, but they have their challenges. One big issue is keeping the antibody-antigen reactions consistent. Things like temperature changes or other substances can affect how well these reactions work, making the biosensors less reliable in different settings. This means they must be kept under strict conditions, which isn’t always accessible outside of a lab.
Another problem is that sometimes antibodies might stick to things, leading to wrong results. This is especially tricky when dealing with complex samples like blood, where lots of different substances are present.
Making these biosensors is also not cheap or simple. Creating antibodies that work well on a sensor’s surface and can still accurately find their target is high-tech work. Furthermore, it is harder to use these sensors everywhere, particularly in places with less money for healthcare.
Lastly, with new diseases, antibody biosensors need constant updates. This means always looking for new targets and tweaking the sensors to detect them, which adds to the challenge. Despite these hurdles, scientists are working hard to make antibody biosensors better and more adaptable for the future.
7. Future Prospective
The rapidly advancing field of scaffold technology and the research of nanomaterials present enormous promise for the future of electrochemical antibody biosensor technology. These developments are poised to significantly elevate biosensors’ sensitivity, specificity, and overall efficiency, particularly in the field of pathogen detection. As we project into the future, four key areas emerge as pivotal to the evolution of this technology, each carrying the potential to revolutionize how we detect and manage bacterial pathogens, thereby enhancing healthcare outcomes and bolstering environmental safety.
Firstly, the continued innovation in nanomaterials and scaffold designs promises to break new ground in biosensor performance. Nanotechnology, with its ability to manipulate materials at the atomic or molecular level, offers unprecedented opportunities to refine the sensitivity and specificity of biosensors. By harnessing the unique properties of nanomaterials such as graphene, gold nanoparticles, and quantum dots, future biosensors will be capable of detecting pathogens at lower concentrations and with greater accuracy. This precision is critical for early detection of diseases, allowing for timely intervention and improved patient outcomes.
Secondly, the integration of digital technologies with biosensor platforms is set to transform the landscape of diagnostics and monitoring. The future envisions biosensors that are fully integrated with smartphones and wearable devices, providing real-time health data and alerts. This digital integration will enable continuous monitoring of health conditions, environmental pollutants, and potential bioterrorism threats, making it easier to respond swiftly to emerging health crises. The accessibility and convenience of such technology will democratize health monitoring, making advanced diagnostics available to a broader population.
Thirdly, advancements in bioengineering and synthetic biology are expected to enhance the selectivity and stability of biosensors. Future research may focus on developing synthetic antibodies or bioreceptors affinity for specific pathogens, improving the biosensors’ ability to discriminate between closely related strains. This specificity is particularly crucial in diagnosing infections with multiple pathogens presenting similar symptoms. Additionally, synthetic biology could offer solutions to the stability issues of biological components, extending the shelf life and usability of biosensors in various settings.
Lastly, the miniaturization and portability will likely continue, aiming to make biosensors more accessible and user-friendly. Future developments could lead to compact, disposable biosensors for single-use applications or highly portable devices for fieldwork, enabling rapid diagnostics and decision-making in remote or resource-limited environments. This miniaturization, coupled with the potential for mass production, could significantly reduce costs and barriers to access, making high-quality diagnostics a reality in low-income regions.
In conclusion, the future of electrochemical antibody biosensor technology is bright, with scaffold technology and nanomaterials playing a central role in shaping its trajectory. By overcoming current limitations and leveraging the potential of these advancements, the next generation of biosensors will offer enhanced capabilities for detecting pathogenic bacteria, fundamentally changing the landscape of healthcare diagnostics and environmental monitoring. This progress promises not only to improve public health outcomes but also to expand the boundaries of what is possible in biosensor technology, heralding a new era of precision and accessibility in diagnostics.
8. Refrences
[1] https://www.bmglabtech.com/en [2] Mikuła, E., Silva, C.E., Kopera, E., Zdanowski, K., Radecki, J. and Radecka, H., 2018. Highly sensitive electrochemical biosensor based on redox-active monolayer for detection of anti-hemagglutinin antibodies against swine-origin influenza virus H1N1 in sera of vaccinated mice. BMC veterinary research, 14, pp.1-9. DOI: 10.1186/s12917-018-1668-9 [3] Mahshid, S.S., Mahshid, S., Vallée-Bélisle, A. and Kelley, S.O., 2019. Peptide-mediated electrochemical steric hindrance assay for one-step detection of HIV antibodies. Analytical chemistry, 91(8), pp.4943-4947. DOI:10.1021/acs.analchem.9b00648 [4] https://www.creative-biolabs.com/Antibody-Analysis-ServicesPeople also visited:
Must-Reads for Job Seekers
Cademix Career Autopilot Plus: Roadmap to Study and Work in Europe
The Career Autopilot Plus program offered by Cademix Institute of Technology Austria provides international job seekers with a comprehensive roadmap…
Cademix Career Autopilot Transformer: Acceleration Program and Support for Career Transformation
The Cademix Career Autopilot Transformer is a comprehensive acceleration program that offers international job seekers a robust roadmap for career…
Career Autopilot
Career Autopilot is the best in Class Career Acceleration and EU Job Placement Program available for international Job-seekers, graduates &…
Tech Career Acceleration Program
Tech Career Acceleration Program – A Training & Coaching program that boosts your employability – A perfect bridge to move…
Cademix Career Center
Cademix Career Center helps jobseekers, graduates, and students to explore personalized roadmaps and pathways companonships to pursue their unique goals….