This article provides a critical examination of EU-funded quantum photonics projects, analyzing how European policy frameworks, strategic directives, and organized funding mechanisms shape research agendas and collaboration models. Focusing on the interplay between large-scale initiatives, skill-building efforts, and innovation roadmaps, it identifies systemic challenges related to project selection, coordination, and assessment. By exploring organizational cultures, educational gaps, and market uncertainties, it underscores the importance of carefully designed strategies that enable sustainable skill development, foster interdisciplinary communication, and enhance the scalability of quantum photonic technologies. The analysis concludes by suggesting pathways toward a more cohesive, transparent, and adaptable funding landscape that better aligns with Europe’s long-term scientific, economic, and societal objectives.
Table of Contents
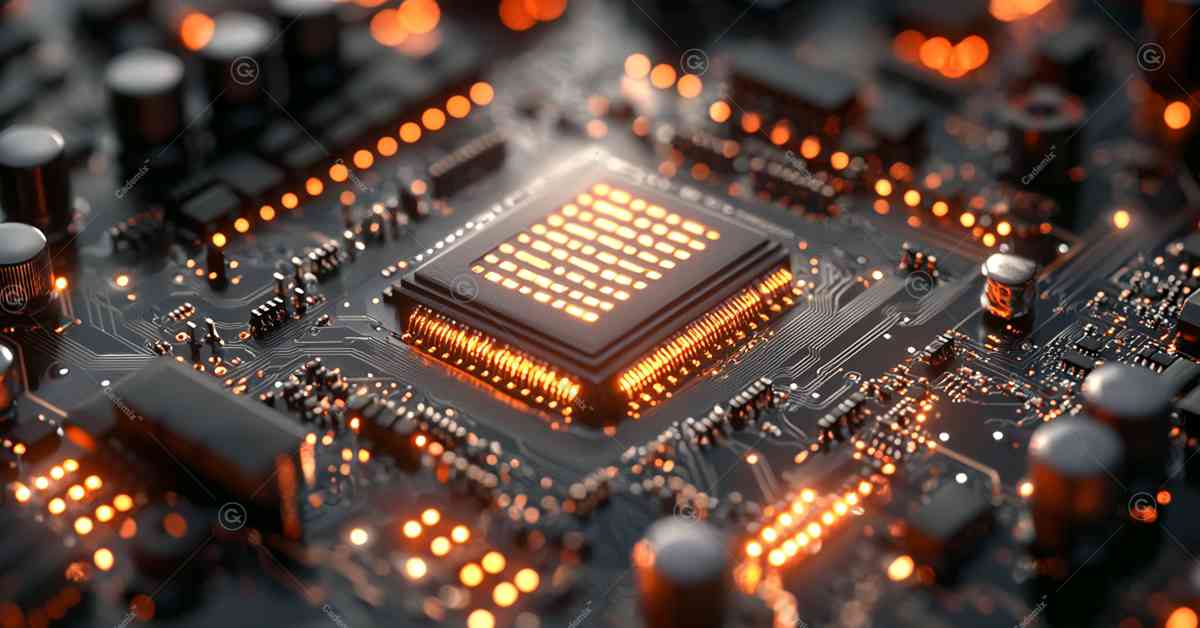
Introduction
The European Union’s investment in quantum photonics embodies a vision that extends far beyond academic curiosity, encompassing industrial innovation, technological sovereignty, and the pursuit of strategic autonomy. Quantum photonics, as part of the broader quantum technologies agenda, promises advances in secure communication, high-precision sensing, and computational capabilities that surpass classical limits. In this context, EU-funded quantum photonics projects are not isolated endeavors; rather, they are nodes within a complex ecosystem of policy directives, research funding frameworks, and educational initiatives that aim to bolster Europe’s capacity for scientific excellence and competitive growth.
Yet, despite the strategic importance of these projects, the process of evaluating their effectiveness and alignment with overarching innovation roadmaps remains a nuanced challenge. Funding instruments such as Horizon Europe and the Quantum Flagship set ambitious objectives, but translating these into tangible outcomes often requires reconciling conflicting priorities, balancing short-term deliverables against long-term aims, and developing skill sets attuned to emergent fields. The landscape is further complicated by the interplay of organizational cultures, national interests, and industry-academia collaborations that do not always align neatly with policy narratives.
This article offers a critical overview of how EU-funded quantum photonics projects operate within this dynamic environment. It examines the factors that influence project selection, design, and assessment, while paying special attention to the underlying policy and strategy frameworks that guide them. Rather than endorsing a particular solution, the discussion highlights structural barriers and systemic tensions, illuminating where improvements might be needed. The goal is to provide insights for policymakers, researchers, educators, and industry stakeholders who seek to optimize the returns on Europe’s significant public investment in quantum photonics, ensuring that breakthroughs emerge not by chance, but through deliberate, well-coordinated efforts.
The Policy Landscape: From Grand Visions to Operational Realities
The European Union’s quantum photonics policy environment is built on a series of official communications, legislative instruments, and strategic documents. High-level initiatives such as the European Quantum Flagship and the EU’s broader Digital Decade agenda articulate a commitment to leading-edge quantum technologies. However, the distance between policy vision and operational reality can be vast. Policymakers often frame the importance of quantum photonics in terms of economic competitiveness, strategic autonomy, and societal benefit, but translating these aspirations into effective calls for proposals, evaluation criteria, and funding mechanisms requires careful navigation.
Indeed, one challenge lies in ensuring that these policy directives remain sufficiently flexible to accommodate the rapid scientific and technological changes characteristic of quantum photonics. Setting too rigid a roadmap risks locking resources into approaches that may become obsolete. Conversely, excessive flexibility might result in diffuse efforts that fail to achieve critical mass. Balancing these extremes demands a nuanced strategy, one that aligns funding calls with emerging research directions, encourages collaboration across borders and disciplines, and remains open to mid-course adjustments based on results and feedback from stakeholders.
Another complexity arises from the multiplicity of actors involved. Policy frameworks may advocate for synergy, but coordination between diverse funding bodies, national agencies, and thematic initiatives can be uneven. Projects might struggle to navigate overlapping calls, conflicting timelines, or contradictory mandates. In some cases, bureaucratic overheads hinder timely project launches or introduce uncertainties that deter prospective participants. As a result, while the EU provides a structured environment to promote quantum photonics, the actual implementation depends heavily on effective inter-institutional communication, standardized evaluation procedures, and the capacity of program managers to interpret and apply high-level directives in a consistent manner.
Research Funding Mechanisms and Their Structural Limitations
EU-funded quantum photonics projects often fall under competitive grant schemes that reward excellence, ambition, and collaboration. Programs like Horizon Europe’s Pillar II or the Quantum Flagship’s thematic calls seek to attract consortia comprising academic institutions, research centers, and companies from multiple EU member states. Although these funding instruments provide substantial resources and encourage interdisciplinary thinking, they also expose structural limitations. The competitive nature of the calls can perpetuate inequality, as well-established institutions with robust administrative support and established networks may have an advantage over less-resourced entities.
The evaluation process itself, typically involving independent expert panels, must grapple with metrics that are not always well-suited to quantum photonics. Assessing a proposal’s feasibility, impact, and innovation level requires knowledge that spans quantum mechanics, semiconductor processing, optical systems, and emerging application domains. Identifying evaluators who can appreciate the intricacies of quantum-enhanced cryptography or integrated photonic chips for quantum computing can be challenging. Consequently, the risk of misalignment between evaluators’ expertise and project content increases, potentially skewing funding decisions and limiting the diversity of funded initiatives.
Time-to-grant periods, reporting obligations, and administrative burdens further complicate the funding landscape. Researchers are often forced to invest significant effort in writing proposals, meeting deliverables, and maintaining compliance with regulations. This administrative load can reduce time spent on actual research, thereby diluting the overall efficiency of the funding system. While accountability and transparency are essential, there is an ongoing debate about finding the right balance between necessary oversight and enabling researchers and innovators to focus on core technological advances.
Skill-Building and Educational Gaps
One of the central tensions in EU-funded quantum photonics projects involves the need to develop a workforce capable of driving innovation and translating research outcomes into marketable products. Skill-building initiatives and educational programs are vital, yet the current landscape reveals significant gaps. Emerging quantum photonics technologies demand competencies that span fundamental physics, materials science, integrated photonics design, advanced simulation tools, and high-precision fabrication techniques. In addition, entrepreneurial, management, and communication skills are increasingly important, given the multidisciplinary, market-facing nature of these projects.
Universities and research institutions are often at the forefront of training early-career researchers, but their curricula may lag behind the rapid developments in quantum photonics. The complexity of setting up comprehensive programs that combine theoretical knowledge with hands-on laboratory experience and industry placements cannot be overstated. Funding agencies and policymakers may call for skill-building, yet the link between project-level training activities and broader educational frameworks is not always well-defined. As a result, some EU-funded projects produce highly specialized skills that remain siloed within a single consortium, rather than contributing to a durable, widely accessible skill base across Europe.
Another complication arises from geographical discrepancies in educational and research infrastructures. While certain member states boast world-class facilities, others face a scarcity of advanced laboratories, experienced faculty, or robust industry linkages. Without addressing these regional imbalances, even the best-funded quantum photonics projects may struggle to elevate the skill level uniformly across the continent. Hence, skill-building must move beyond aspirational rhetoric, translating into policy measures that promote mobility, resource sharing, and standardized certification schemes that can guide learners and employers alike.
Organizational Cultures and Interdisciplinary Communication
Quantum photonics thrives at the intersection of disciplines. Physicists, material scientists, engineers, computer scientists, and entrepreneurs must work together to design, fabricate, and test complex photonic chips that encode and manipulate quantum states. This interdisciplinary dimension can enrich EU-funded projects, but it also introduces cultural and communication challenges. Different fields employ distinct jargon, research methodologies, and validation criteria. Without careful mediation, misunderstandings may arise, slowing progress and weakening the coherence of project goals.
The organizational cultures of participating entities can further complicate matters. Academic institutions prioritize fundamental research and publication records, while industry partners focus on commercialization, intellectual property management, and time-to-market. Policy directives may encourage synergy, yet the practical task of reconciling these motivations and managing expectations falls on project coordinators and consortium leaders. Some EU-funded projects struggle to maintain a cohesive identity, encountering tensions over milestone definitions, data sharing protocols, or the dissemination of intermediate results.
Funding bodies and policymakers often highlight the importance of interdisciplinary dialogue, but providing the frameworks and incentives for effective communication remains a work in progress. Workshops, training sessions, and dedicated platforms for knowledge exchange can help, though their success depends on genuine buy-in from participants and the willingness of senior researchers and managers to embrace new organizational models. Overcoming these cultural barriers is essential to ensure that EU-funded quantum photonics projects do not become fragmented clusters of expertise, but rather integrated endeavors that yield transformative results.
Market Uncertainties and the Path to Commercialization
EU-funded quantum photonics projects frequently operate with an eye toward future markets, whether in secure communications, sensing applications, or next-generation computing architectures. However, the market landscape for quantum photonics remains uncertain, influenced by evolving standards, regulatory frameworks, and competing technological paradigms. Anticipating these shifts is challenging, and some projects may design solutions for market conditions that do not materialize as expected.
This uncertainty poses strategic dilemmas for project consortia. On one hand, embracing a strong market orientation early on could direct research toward practical outcomes and engage industrial partners who can later leverage results. On the other hand, focusing too heavily on near-term market applications risks constraining the fundamental research dimension, limiting the exploration of unconventional ideas that might yield disruptive innovations down the line. The dual nature of quantum photonics—as both a fundamental scientific pursuit and an industrial development opportunity—forces stakeholders to navigate a delicate path.
EU policy guidelines and innovation roadmaps encourage projects to consider impact pathways and technology readiness levels. While these guidelines help shape strategic thinking, their execution can feel prescriptive. Some researchers argue that not all transformative breakthroughs can be foreseen or framed in terms of immediate market viability. Striking a balance between exploratory research and well-defined commercial endpoints is an ongoing challenge. Projects that find a nuanced approach, preparing for multiple market scenarios while maintaining a core commitment to scientific rigor, stand a better chance of long-term relevance.
The Role of International Collaboration and Global Competition
The EU’s quantum photonics agenda unfolds against a backdrop of global competition. Regions such as North America and East Asia also invest heavily in quantum technologies, fostering their own ecosystems and forging international partnerships. EU-funded projects must acknowledge that scientific and market leadership is not guaranteed. Instead, Europe’s strength often lies in its collaborative ethos, diverse talent pools, and established frameworks for cross-border research funding.
International collaboration can enhance the quality of EU-funded quantum photonics projects by broadening perspectives, introducing complementary expertise, and connecting European consortia to global supply chains. However, global engagement also raises strategic questions. Intellectual property issues, export controls, and differing regulatory norms can complicate partnerships. If EU policies do not offer clear guidelines or safeguard mechanisms, projects may inadvertently expose sensitive findings or rely on external supply chains that undercut Europe’s strategic autonomy.
Moreover, global competition accentuates the need for EU-funded projects to benchmark their progress and performance against international standards. Without transparent evaluation metrics and comparative analyses, it becomes difficult to determine whether Europe is keeping pace. Implementing robust benchmarking exercises and publishing comparable data could stimulate healthy competition among project consortia, motivating them to refine their approaches and continually push the limits of what quantum photonics can achieve.
Cultural and Psychological Aspects of Funding Allocation
Beneath the technical and strategic discussions lies a human dimension. Researchers, engineers, and project managers operate within a psychological environment shaped by funding uncertainties, career pressures, and the high-stakes nature of emerging quantum fields. Securing an EU-funded project can bring prestige, job security for junior researchers, and the resources needed to realize ambitious ideas. Yet, the competitive funding climate also fuels stress, encourages risk-aversion, and may incentivize the over-promotion of results to meet evaluators’ expectations.
When careers hinge on grant success, there is a risk of conforming to established paradigms, choosing “safe” approaches that match evaluation panel preferences rather than pursuing bold new directions. This dynamic can stifle creativity and slow the discovery of truly disruptive photonic technologies. Policymakers and funding agencies, while understandably focused on accountability and demonstrable impact, must consider how their criteria shape the research culture. Developing mechanisms that reward long-term thinking, acknowledge the value of negative results, and foster stable career paths could reduce psychological strain and encourage more genuine scientific risk-taking.
Strengthening dialogue between funders and the research community can help recalibrate expectations. Workshops, feedback sessions, and advisory boards that include researchers of various career stages and institutional backgrounds can provide insights into how selection criteria influence behavior. By understanding these cultural and psychological aspects, policymakers can design funding instruments that promote a healthier, more balanced ecosystem conducive to innovation in quantum photonics.
The Importance of Transparent and Adaptive Evaluation Processes
Effective evaluation is at the heart of EU-funded quantum photonics projects. Without clear, transparent, and adaptive assessment mechanisms, it becomes difficult to identify best practices, scale up successes, or learn from failures. Evaluation should consider not only scientific achievements, but also the ability of projects to foster skill-building, communication, and long-term strategic vision. Yet, as mentioned, the complexity of quantum photonics complicates traditional metrics of success.
One approach to improving evaluation involves embracing more holistic criteria. Instead of focusing solely on deliverables and publications, evaluation panels could weigh contributions to community-building, training activities, standard-setting, or the establishment of sustainable infrastructures like shared testing facilities. A project that advances metrology standards for photon sources or integrates novel education modules into an engineering curriculum may provide lasting benefits that extend beyond a single research result.
Adaptive evaluation also means that funding bodies should revisit assessment frameworks periodically, adjusting criteria as the field evolves. Quantum photonics today may have different priorities than it will five years from now, as certain concepts mature and others fade. By continuously refining evaluation methods, policymakers can ensure that funded projects remain aligned with Europe’s shifting innovation landscapes and that resources are directed toward those areas most capable of yielding genuine progress.
Revisiting Educational Strategies and Skill-Building Approaches
Given that skill-building emerged as a major area of concern, it merits closer scrutiny. The slow pace of curriculum reform and the inherent inertia in academic systems mean that bridging the gap between project-specific training and broader educational frameworks will not happen overnight. However, acknowledging this gap is the first step toward addressing it. Policymakers could, for instance, incentivize projects to collaborate with educational institutions in developing new courses, online modules, or certification programs that can outlive the grant period.
Another potential solution involves establishing European graduate schools or training centers focused on quantum photonics. By pooling resources from several projects and engaging with industry stakeholders, these centers could offer structured programs that blend theory and practice. The existence of shared facilities, internships with industrial partners, and mentorship from experienced scientists could encourage a more fluid exchange of knowledge and skills. Additionally, using digital platforms to disseminate lecture recordings, lab demonstrations, and research seminars could democratize access to high-quality training materials, reducing the disparity among regions.
Ultimately, any strategy to enhance skill-building must recognize that not all learners have the same background or access to resources. Tailored efforts, such as scholarships for underrepresented groups or special support for researchers from countries with less-developed infrastructures, can help ensure that the benefits of EU-funded quantum photonics extend across social and geographical boundaries. By committing to more inclusive education policies, the EU can foster a talent pipeline that fully exploits the continent’s intellectual diversity.
Strengthening Policy-Research Dialogue and Ecosystem Cohesion
One theme consistently emerging is the necessity for stronger dialogue and cohesion between policymakers, researchers, educators, and industry representatives. Although formal consultations, advisory boards, and stakeholder meetings exist, their impact varies. Some participants may feel that their insights vanish into policy documents without influencing real funding criteria or strategic choices. Others may perceive that calls for proposals are pre-determined, leaving little room for genuine debate about priorities.
To address these concerns, more transparent and iterative consultation processes can be instituted. Allowing stakeholders to comment on draft calls, provide input on evaluation criteria, or suggest modifications to skill-building initiatives ensures that policymaking is not a top-down affair. Embracing more iterative design cycles, where smaller pilot projects inform subsequent large-scale calls, could also improve alignment. By learning from incremental experiments, policymakers can adjust funding mechanisms, criteria, or educational guidelines before scaling them up, reducing the risk of systemic inefficiencies.
Strengthening ecosystem cohesion also implies clarifying the division of labor among various funding bodies and agencies. A more integrated approach could see the European Commission, national funding agencies, and private foundations coordinating their efforts, reducing redundancies and gaps. When skill-building, research, and commercialization support form a coherent continuum, projects gain a more stable footing, enabling them to progress naturally from fundamental investigations to demonstrators and, eventually, viable products.
Policy Innovations, Pilot Experiments, and Continuous Improvement
The path forward for EU-funded quantum photonics projects involves embracing policy innovations and learning from pilot experiments. Incremental reforms, such as introducing new evaluation rubrics or incentivizing open science practices, could gradually reshape the research culture. Larger-scale experiments, like creating specialized quantum photonics testbeds accessible to multiple projects, could accelerate technology validation and reduce the duplication of efforts.
Moreover, policy strategies should remain adaptable. As quantum photonics matures, certain bottlenecks may vanish while others emerge. Maintaining flexible funding envelopes that can be redirected to address unexpected challenges, such as a sudden shortage of skilled workforce or a supply chain disruption, ensures resilience. Continuous improvement also depends on monitoring the long-term fate of funded projects. Tracking how results translate into patents, spin-offs, standardization contributions, or international partnerships offers feedback loops essential for refining future calls.
If the EU commits to a culture of learning and adaptation, it can transform the complexity of quantum photonics into a strategic advantage. By recognizing that the journey from fundamental discovery to market dominance requires more than just money—demanding policy intelligence, educational foresight, organizational empathy, and cross-cultural communication—Europe positions itself as a leader not just in quantum technologies, but in innovation governance more broadly.
Conclusion and Call to Action
Evaluating EU-funded quantum photonics projects reveals a landscape of promise and tension. On one hand, significant resources and visionary policies have created fertile ground for breakthroughs in secure communication, ultra-precise sensing, and unprecedented computational power. On the other, structural imbalances, administrative complexities, skill-building gaps, cultural misunderstandings, and market uncertainties temper expectations. The challenge now is not merely to fund more projects, but to ensure that these projects operate within a refined ecosystem that nurtures scientific excellence, strategic thinking, and social responsibility.
The call to action is clear: policymakers must refine evaluation criteria, support flexible and transparent consultations, and embrace iterative experimentation with new funding models. Educational stakeholders should push for more integrative curricula, online resources, and collaborative training centers that equip the next generation with a comprehensive skill set. Industry and academia must learn to speak each other’s language, bridging cultural divides and aligning objectives with a shared vision of Europe’s quantum future.
If these collective steps are taken, EU-funded quantum photonics projects can transition from scattered efforts to coordinated achievements, forging robust innovation roadmaps that endure beyond political cycles and market fluctuations. By acknowledging challenges candidly and approaching them with strategic foresight and cultural sensitivity, Europe can harness the full potential of quantum photonics, ensuring that its investments yield not only scientific results but also long-lasting economic, educational, and societal benefits.
References and Further Reading
European Commission. (2020). Horizon Europe: The next research and innovation framework programme. https://ec.europa.eu/info/horizon-europe_en
European Quantum Flagship. (2021). Strategic Research Agenda for Quantum Technologies. https://qt.eu/roadmap
Flamini, F., Spagnolo, N., & Sciarrino, F. (2018). Photonic quantum information processing: a review. Reports on Progress in Physics, 82(1), 016001. https://doi.org/10.1088/1361-6633/aad5b2
Nielsen, M. A., & Chuang, I. L. (2010). Quantum Computation and Quantum Information: 10th Anniversary Edition. Cambridge University Press. https://doi.org/10.1017/CBO9780511976667
Perez, D., Gasulla, I., & Capmany, J. (2017). Field-programmable photonic arrays. Optics Express, 25(3), 282–294. https://doi.org/10.1364/OE.25.000282
Politi, A., Matthews, J. C. F., Thompson, M. G., & O’Brien, J. L. (2009). Integrated quantum photonics. IEEE Journal of Selected Topics in Quantum Electronics, 15(6), 1673–1684. https://doi.org/10.1109/JSTQE.2009.2026060
Soref, R. (2010). The past, present, and future of silicon photonics. IEEE Journal of Selected Topics in Quantum Electronics, 16(1), 167–176. https://doi.org/10.1109/JSTQE.2009.2035196
Wang, J., et al. (2018). Multidimensional quantum entanglement with large-scale integrated optics. Science, 360(6386), 285–291. https://doi.org/10.1126/science.aar7053
Xu, X., et al. (2020). Photonic inverse design: From fundamentals to applications. Advanced Optics and Photonics, 12(3), 828–887. https://doi.org/10.1364/AOP.381966
Zhang, H., et al. (2021). Integrated quantum photonics: Current status and future prospects. APL Photonics, 6(7), 071101. https://doi.org/10.1063/5.0045024